|
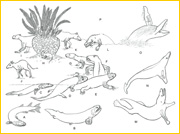 |
The Iconology of a Humerus Tale
by Farish Jenkins |
|
|
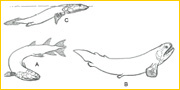 |
~A,
B, C~
By Late
Devonian times (~360 million years ago, mya), the rudimentary design
of tetrapod limbs was established among sarcopterygian fishes that
are known from diverse fossils from North America, Australia, eastern
and western Europe. Only a few sarcopterygians survive today--lungfishes,
with a species each in Africa, Australia and South America, and
several species of the coelacanth Latimeria in the coastal waters
of Africa and Indonesia.
Although
the humerus began as a simple element, flattened and block-like,
a few features that are primitively present (an entepicondylar process,
a ventral crest) persist throughout the evolutionary diversification
of tetrapods. Thus the entepicondyle and deltopectoral crest of
humans are piscine features. All sarcopterygians possessed bones
that are homologous with radius and ulna. Few groups, however, had
distal limb elements that are prototypic of digital rays (B, C).
And only one group, the elpistostegids (C), not only had protodigits
but also an array of cranial features antecedant to the tetrapod
condition.1-7
|
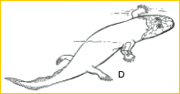 |
~D~
Acanthostega,
retaining gills but with terrestrial limbs, is one of a number of
primitive amphibians known from Late Devonian-Early Carboniferous
deposits in Australia, eastern Europe, Greenland and North America.
A flexed elbow supported the trunk, and the entepicondylar process
enlarged to accommodate powerful antebrachial flexors. But the number
of fingers remained distinctively fishy.8-9
|
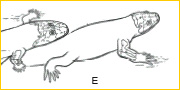 |
~E~
Humeral
robustness and complexity increased as amphibians diversified in
terrestrial habitats during the Late Paleozoic, and pentadactyly
emerged as the tetrapod standard.10
|
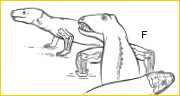 |
~F~
By the
Late Carboniferous (~310 mya), the ancestral stocks of modern amniotes
appeared, possessing in common the reproductive novelty of extraembryonic
membranes– the amnion, chorion, and allantois. One primitive
lineage, the sprawling pelycosaurs, evolved into both carnivorous
and herbivorous niches. The humerus in predatory sphenacodontid
pelycosaurs was of complex form, attesting to a differentiation
of limb musculature and diversity of limb use.11
|
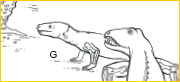 |
~G~
Therapsids,
or mammal-like reptiles, descended from pelycosaurs and flourished
during Permian and Triassic times (290-215 mya). Cynodonts known
from South Africa and South America are evolutionarily the most
advanced therapsids. In numerous dental, cranial, axial and appendicular
features, cynodonts acquired anatomical patterns antecedent to the
mammalian bauplan- including an upright, adducted limb posture and
a prototypically mammalian humerus.12
|
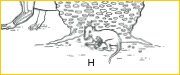 |
~H~
By the
Late Triassic (~ 210 mya), true mammals appeared. Tiny in size,
they remained for the most part diminutive throughout the remainder
of the Mesozoic in a world dominated by dinosaurs and other reptiles.13
|
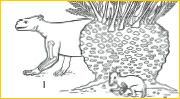 |
~I~
Substantial
diversification of mammals began after the widespread extinctions
at the close of the Cretaceous (65 mya). Among Paleocene mammals,
condylarths represent some incipient specializations related to
herbivory. Phenacodus possessed a bunodont dentition for browsing,
and in place of claws, broad unguals functioned as proto-hooves.
The robust skeletons of these heavy bodied, archaic forms reveal
little of the cursorial adaptations developed by modern ungulates.14,15
|
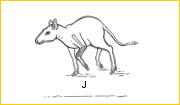 |
~J~
Among
many groups of mammalian herbivores that have evolved, artiodactyls
– or even-toed ungulates – are among the most successful
in terms of taxonomic diversification. Diacodexis of the Early Eocene
(55 mya) is the earliest known artiodactyl, close to the common
ancestry of mouse deer, musk deer, deer, camels, pigs, hippos, giraffes,
cows and antelopes. All artiodactyls share a distinctive, unique
feature. The talus bears two trochleae; in addition to the conventional
proximal trochlea of the tibio-talar joint, the talar head (articulating
with the navicular) is also trochlear in form. This ‘double-pulleyed’
talus is part of a spring mechanism that contributes to the prodigious
running and bounding abilities of many artiodactyls.16
|
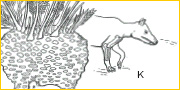 |
~K~
Elomeryx,
an anthracotheriid artiodactyl from the Late Eocene through Late
Oligocene of Europe and North America (38–23 mya), is placed
in the superfamily Hippopotamoidea, but possesses premolars that
are suggestively similar in form to those of the earliest known
whales.17,18
|
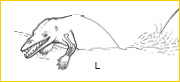 |
~L~
Along
the shores of the once vast Tethys Sea (of which the present day
Mediterranean is but a remnant), primitive whales appeared during
the Eocene. First recognized from fragmentary skulls, recent finds
in Pakistan have turned up vertebrae and limbs. The hindfeet of
these amphibious archaeocetes had a talus with two trochleae --
whales are derived artiodactyls!19-21
|
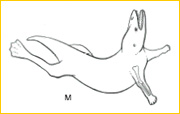 |
~M~
Rhodocetus,
a primitive archaeocete cetacean found in Eocene deposits of Pakistan,
swam by paddling with powerful hind limbs.21
|
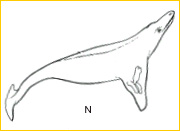 |
~N~
Durodon,
an advanced basilosaurid cetacean known from Late Eocene sediments
in Egypt, abandoned hind limbs in favor of flexing and extending
the caudal fluke, a mechanism employed by all modern whales.22
|
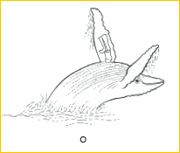 |
~O~
A humpback
whale, Megaptera novaeangliae (‘the big wing of New England’),
breaches with an exuberant back flip in Cape Cod coastal waters.
As with so many other mammals, whales are social and communicative,
but have otherwise transformed every other aspect of their mammalian
heritage into an elegant, perfected return to the sea. In some,
teeth have been abandoned in favor of keratinous sheets of baleen,
sieving plankton from 45,000-liter gulps of water or benthic life
from 2-20 m2 mouthfuls of mud and sand sucked from the oceanic floor.
Yet the humerus has reverted to a simple structural form, as in
their sarcopterygian ancestors of the Devonian.
|
|
|
~References~
- Westoll,
T. S. 1943. The origin of the primitive tetrapod limb. Proceedings
of the Royal Society of London B, 131:373-393.
- Andrews, S. M. and T. S. Westoll. 1970. The postcranial skeleton
of Eusthenopteron foordi Whiteaves. Transactions of the Royal Society of Edinburgh,
68:207-239.
- Jarvik, E. 1980. Basic Structure and Evolution of Vertebrates.
Vols. 1 and 2. New York:Academic Press.
- Andrews, S. M. 1985. Rhizodont crossopterygian fish from the Dinantian
of Foulden, Berwickshire, Scotland, with a re-evaluation of the group. Transactions
of the Royal Society of Edinburg, 76:67-95.
- Vorobyeva, E. and H.-P. Schultze, 1991. Decription and systematics
of panderichthyid fishes with comments on their relationship to tetrapods, pp. 68-109.
In Origins of the Higher Groups of Tetrapods: Controversy and Consensus (H.-P. Schultze
and L. Trueb, eds.). Comstock Press:Ithaca.
- Daeschler, E. B. and N. H. Shubin. 1998. Fish with fingers? Nature,
391:133.
- Daeschler, E. B., N. H. Shubin and F. A. Jenkins, Jr. 2004. A new
member of the sister group of tetrapods: an elpistostegid fish (Sarcopterygii, Elpistostegalia)
from the Fram Formation, Ellesmere Island, Nunavut Territory, Canada. Journal
of Vertebrate Paleontology, 24:50A.
- Coates, M. I. 1996. The Devonian tetrapod Acanthostega gunnari
Jarvik: postcranial anatomy, basal tetrapod relationships and patterns of skeletal evolution.
Transactions of the Royal Society of Edinburgh, 87:363-421
- Clack, J. A. 2002. Gaining Ground: The Origin and Evolution of
Tetrapods. Bloomington:Indiana University Press. ix + 369 pp.
- White, T. E. 1939. Osteology of Seymouria baylorensis Broili.
Bulletin of the Museum
of Comparative Zoology, Harvard University, 85:325-409.
- Romer, A. S. and L. W. Price. 1940. Review of the Pelycosauria.
Geological Society of
America Special Papers, 28:1-538.
- Jenkins, F. A., Jr. 1971. The postcranial skeleton of African
cynodonts: problems in
the early evolution of the mammalian postcranial skeleton. Bulletin
of the Yale
Peabody Museum, 36:1-216.
- Jenkins, F. A., Jr., and F. R. Parrington, F.R.S. 1976. The postcranial
skeletons of the
Triassic mammals Eozostrodon, Megazostrodon and Erythrotherium. Philosophical
Transactions of The Royal Society of London B, 273:387-431.
- Osborn, H. F. 1910. The Age of Mammals in Europe, Asia and North
America. New
York:The Macmillan Company. xvii + 635.
- Archibald, J. D. 1998. Archaic ungulates (“Condylartha”),
pp. 292-329. In Evolution
of Tertiary Mammals of North America (C. M. Janis, K. M. Scott and
L. L. Jacobs,
eds.). Cambridge:Cambridge University Press.
- Rose, K. D. 1982. Skeleton of the earliest known artiodactyl.
Science, 216:621-623.
- Scott, W. B. 1940. Part IV: Artiodactyla. In The Mammalian Fauna
of the White River
Oligocene (W. B. Scott and G. L. Jepsen, eds.). Transactions of the
American
Philosophical Society, N.S., 28:363-746.
- Kron, D. G. and E. Manning. 1998. Anthracotheriidae, pp. 381-388.
In Evolution
of Tertiary Mammals of North America (C. M. Janis, K. M. Scott and
L. L. Jacobs,
eds.). Cambridge:Cambridge University Press.
- Gingerich, P. D., N. A. Wells, D. E. Russell, and S. M. Ibrahim
Shah. 1983. Origin of
whales in epicontinental remnant seas: new evidence from the Early
Eocene of
Pakistan. Science, 220:403-406.
- Thewissen, J. G. M., E. M. Williams, L. J. Roe, and S. T. Hussain.
2001. Skeletons of
terrestrial cetaceans and the relationship of whales to artiodactyls.
Nature, 413:
277-281.
- Gingerich, P. D., M. ul Haq, I. S. Zalmout, I. H. Khan, and M.
Sadiq Malkani. 2001.
Origin of whales from early artiodactyls: hands and feet of Eocene
Protocetidae
from Pakistan. Science, 293:2239-2242.
- Gingerich, P. D. 2003. Land-to-sea transition in early whales:
evolution of Eocene Archaeoceti (Cetacea) in relation to skeletal proportions and locomotion
of living semiaquatic mammals. Paleobiology, 29:429-454.
FAJJr
Cambridge, January 25, 2005 |
|