Lecture 4:
Unifying chemistry and mechanics: Modeling fracture mechanics of chemically complex materials using reactive force fields
Copy of slides For decades, it has been the dream of computational material scientists to model the complexity of quantum mechanical interactions of atoms in systems comprising of thousands of molecules. So far, such simulations have not been possible. One either had to compromise on system size, as in DFT methods limited to about 100 atoms, or on accuracy and the level of chemistry that can be modeled, as done when using empirical potentials. In this fourth lecture of the series entitled “From nano to macro: Introduction to atomistic modeling techniques”, we present an introduction into a new generation of reactive force fields, focusing on the ReaxFF theory. Reactive force fields represent a significant breakthrough in closing the enormous gap between QM (DFT) and empirical nonreactive potentials, by enabling to model the complexity of chemical reactions, while still being computationally tractable and applicable to systems greater than 1E4 atoms. We review the basic concepts of reactive force fields, and compare the foundations and approaches with classical, nonreactive formulations. We demonstrate that including concepts such as charge flow, energy continuity during chemical reactions and changes of bond orders, as well as shielded nonbonding van der Waals and ionic interactions enables to describe the properties of complex, hierarchical materials with quantum mechanical accuracy.
Reactive force fields find particularly useful applications in modeling the mechanical behaviour of chemically complex materials. This is because the deformation and fracture of materials is governed by the dynamics of breaking and formation of chemical bonds between atoms. When brittle materials break, thousands of cracks propagate as bonds between atoms are broken, generating two newmaterial surfaces. Deformation of ductile materials occurs by motion of crystal defects such as dislocations. In biological materials such as those based on proteins, the microstructure ofmaterials is a highly complex arrangement of different amino acids, forming complicated three-dimensional folded structures. In all of these cases, a correct description of chemistry is essential to provide accurate theoretical models of the deformation and fracture behavior. Examples of applications discussed in this lecture include deformation and fracture of collagen fibers, mechanically stimulated unfolding of proteins, and fracture of metals competing with surrounding chemical elements leading to oxidative processes. We also present recent studies of formation of water molecules from molecular oxygen and hydrogen at a platinum catalyst (see Figure). Reactive potentials allow modeling the realistic dynamics of water formation with thousands of atoms, over time scales of nanoseconds, with quantum mechanics accuracy, but at a cost of the fraction of complete DFT calculations. We conclude with an outlook to future studies and applications of the new modeling techniques.
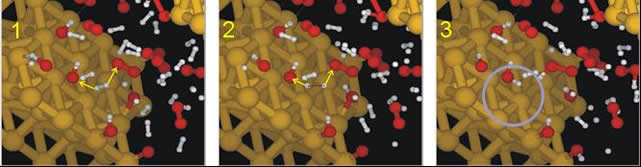
Figure: Model system of formation of water at a Pt surface. Reactive force fields enabel modeling the complexities of such chemical reactions in systems comprising of several thousands of atoms, at time scales of several nanoseconds, with quantum mechanics accuracy but at a fraction of the cost of DFT calculations (see ref. [9]).
Literature and further reading
1. Buehler, M.J., et al., The Computationalmaterials Design Facility (CMDF): A powerful framework for multiparadigm multi-scale simulations. Mat. Res. Soc. Proceedings, 2006. 894 : p. LL3.8. (Link to paper...)
2. Buehler, M.J., et al., Formation of water at a Pt(111) catalyst surface: A study using the ReaxFF reactive force field. Mat. Res. Soc. Proceedings, 2006. 900E : p. O3.9 (Link to paper...)
3. Duin, A.C.T.v., et al., ReaxFF: A Reactive Force Field for Hydrocarbons. J. Phys. Chem. A, 2001. 105 : p. 9396-9409.
4. Nielson, K.D., et al., Development of the ReaxFF reactive force field for describing transition metal catalyzed reactions, with application to the initial stages of the catalytic formation of carbon nanotubes. J. Phys. Chem. A., 2005. 109 : p. 49.
5. Duin, A.C.T.v., et al., ReaxFF SiO: Reactive Force Field for Silicon and Silicon Oxide Systems. J. Phys. Chem. A, 2003. 107 : p. 3803-3811.
6. van Duin , A.C.T. , et al., Application of ReaxFF reactive force fields to transition metal catalyzed nanotube formation. Abstracts Of Papers Of The American Chemical Society, 2004. 227 : p. U1031-U1031.
7. Brenner, D.W., et al., A second-generation reactive empirical bond order (REBO) potential energy expression for hydrocarbons. Journal Of Physics-Condensedmatter, 2002. 14 (4): p. 783-802.
8. Stuart, S.J., A.B. Tutein, and J.A. Harrison, A reactive potential for hydrocarbons with intermolecular interactions. Journal Of Chemical Physics, 2000. 112 (14): p. 6472-6486.
9. Buehler, M.J., A.C.T.v. Duin, and W.A. Goddard, Multi-paradigm multi-scale modeling of dynamical crack propagation in silicon using the ReaxFF reactive force field. Submitted for publication, 2005.
|