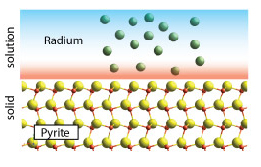
Toxic metals and radionuclides existing in a variety of settings pose serious threats to ecosystems and to human health. Disposal of toxic metals and radioactive wastes generated at DOE facilities has resulted in widespread migration of contaminants into surrounding soils and groundwaters. Further, naturally occurring radionuclides, such as radium, are found in appreciable quantities within produced waters generated from hydraulic fracturing operations. An ongoing interest is to understand the biogeochemical and coupled processes mediating the fate and retention of these constituents within soils, sediments, and natural waters.
The cycling of major and micro-nutrients within terrestrial systems is relevant to an enormous suite of environmental challenges, including water quality, food security, and global climate change. We seek to understand biogeochemistry of micronutrients pertinent to human health, such as iodine, and understand reactions responsible for retention, mobilization, and transformation in terrestrial systems.
The role of sunlight on the degradation and transformation of organic carbon, particularly in terrestrial environments, is important yet currently enigmatic. Exposure to sunlight—even intermittently—is shown to alter the structure of natural organic matter in soil-sedimentary environments, hence altering subsequent processing by microorganisms. Accordingly, we are interested in undertanding the transformative effects of sunlight on the cycling of terrestrial organic matter, and examine causal links between sunlight exposure and enhanced or diminished bioavailability to microorganisms responsible for transforming terrestrial organic matter to greenhouse gases, particularly carbon dioxide and methane.
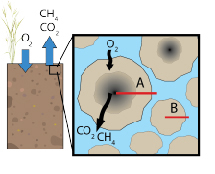
Soils are structured media that have a multitude of pore domains, resulting in varying degrees of advective and diffusive solute and gas transport. A spectrum of biogeochemical processes may therefore function at the aggregate (micron to cm) scale, where the formation of steep chemical gradients is observed. Methane, a potent greenhouse gas, is produced when reducing conditions are prevalent; such conditions are observed within soil and sediment aggregates where microbial consumption of oxygen exceeds the rate of molecular diffusion. Methane may diffuse from the center of soil aggregates to soil macropores, which are conduits to the atmosphere, or be consumed by methanotrophs, which colonize anoxic-oxic boundaries. We are interested in understanding the effects of aggregate geometry on methanogensis and methanotrophy transpiring within soils and sediments, as well as quantifying the linked effects of physical variation and microbial activity responsible for net methane efflux from soils and wetland sediments.
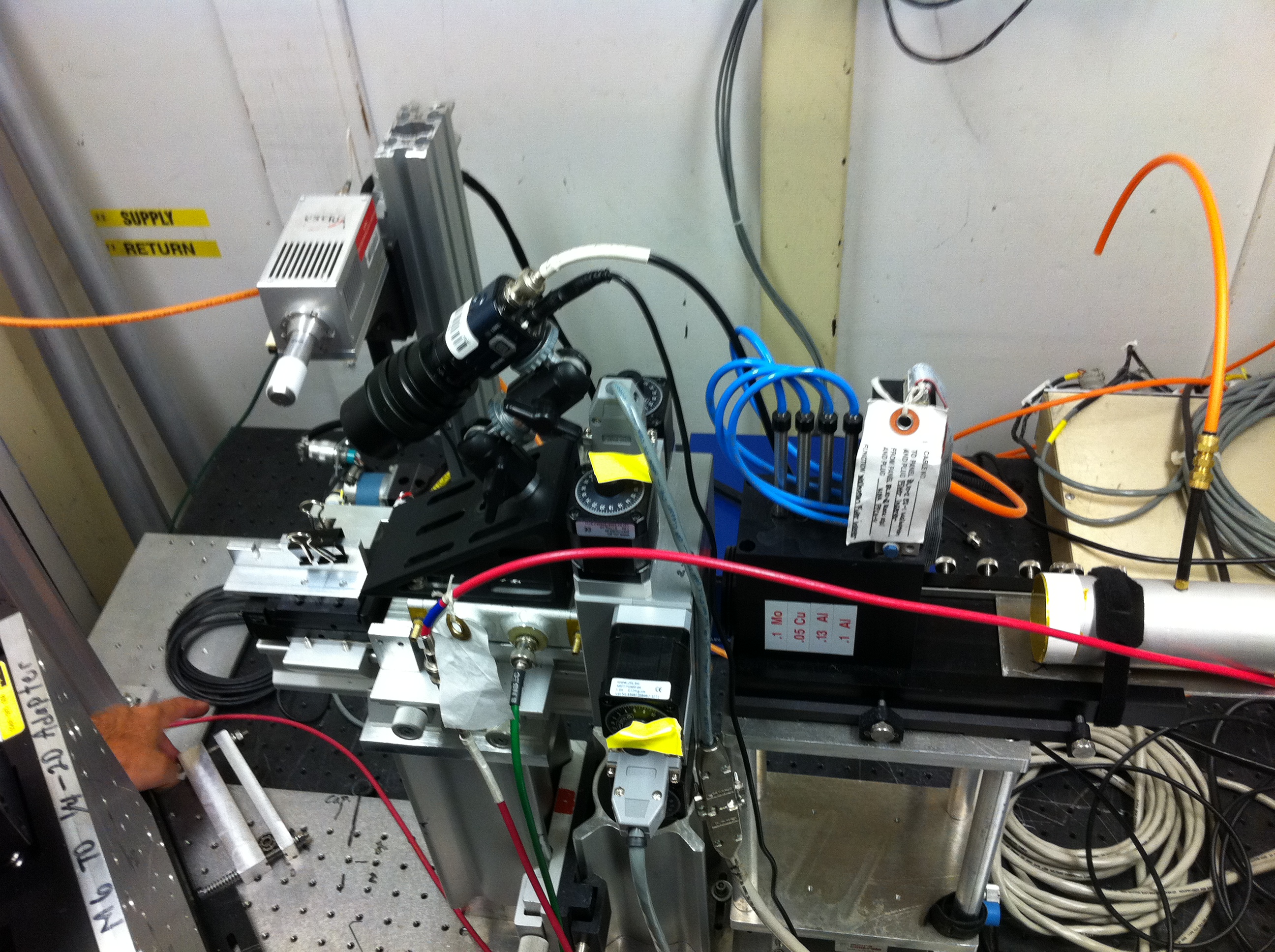
We employ a variety of conventional and sychrotron based methods to analyze samples generated from laboratory and field based studies. In particular, we utilize state of the art spectroscopic and microscopic techniques (e.g. micro and bulk X-ray absorption spectroscopy, micro-fluorescence X-ray tomography, and micro-focused X-ray fluorescence spectroscopy) as well as computational tools (reactive transport modeling) to link molecular and field-scale studies of element cycling.