Workshop
Proceedings: Short Papers
Potential for the use of Remotely Operated Vehicles (ROVs) as a platform
for passive acoustics
Rodney Rountree1, Francis Juanes2, and Joseph E.
Blue3
1Program Manager, Mount Hope Bay Natural Laboratory, School
for Marine Science and Technology, UMASS Dartmouth, 706 Rodney French
Blvd., New Bedford, MA 02744-1221 rrountree@UMassD.Edu
2Department of Natural Resources, University of Massachusetts,
Amherst, MA 01003
3President, Leviathan Legacy, Inc., 3313 Northglen Drive, Orlando,
FL 32806 jblue46498@aol.com
Introduction
We are still largely ignorant of the distribution and behavior of the
great majority of marine fish. Possibly one of the greatest challenges
to researchers attempting to study the behavioral ecology of fishes is
that of finding the fish in the first place. Since some fish are soniferous,
acoustic detection and tracking may offer methods of population assessment
for management decisions. Passive acoustic techniques can be a valuable
tool for the identification of essential fish habitats (EFH) for soniferous
species. These techniques can allow for non-destructive surveys of large
areas to pinpoint habitats frequented by soniferous species, particularly
during spawning events when vocal activity tends to be greatest. Studies
of fish sounds can provide a wealth of data on temporal and spatial distribution
patterns, habitat use, and spawning, feeding, and predator avoidance behaviors.
Currently most investigators use simple omnidirectional hydrophones and
can usually only locate the general area of a spawning aggregation, but
have often been forced to use circumstantial evidence of the identity
and behavior of the calling species (e.g. Saucer and Baltz 1993, Luczkovich
et al. 1999a,b). Attempts to use passive acoustics as a tool to identify
EFH based on spatial patterns in sound production is also critically hampered
by the lack of sufficient data describing the sound characteristics of
individual species and behaviors under field conditions. We propose that
acoustic technologies utilizing hydrophone arrays to home in on sound
sources can greatly improve the study of soniferous fishes and their habitat
requirements. First, homing in on sound sources will provide a valuable
new tool to validate the identity of sound producers, especially when
coupled with underwater photography or video devices. Second, the ability
to home in on vocal fishes would enhance our ability to correlate fish
sound production with specific locations and habitats. In this paper,
we describe our preliminary attempts to develop a Soniferous Fish Locator
(SFL) for use with a remotely operated vehicle (ROV) to home in on fish
sound sources and make recommendations for future efforts.
Tracking and Homing Basics
The use of passive acoustics for homing was developed for naval warfare
during and before WW II. A passive acoustics homing system was implemented
on torpedoes for destruction of ships and submarines. The technique of
homing was extended to detection and tracking of submarines by sonobuoys
during World II. These systems were developed before the advent of small,
fast computers and were implemented with electronics that are now known
as operational amplifiers. Adequate Signal-to-Noise ratios were required
for implementing these techniques. Homing on ships and submarines by torpedoes
requires only 2 directional hydrophones because the torpedo body blocks
out sound from behind it. The available aperture is small so frequencies,
such that there are several wavelengths across the directional hydrophones,
are used for the lowest frequency in the tracking bandwidth. The torpedo
determines the bearing from acoustic signature (signal) of the ship or
submarine by cross-correlating the signatures from the 2 hydrophones.
The cross-correlation function is:
 |
(1)
|
where s1(t) and s2(t) are the noise-free time signals
from the 2 hydrophones, t is the time delay
between the arrivals of the signals at their respective hydrophones and
T is the period over which the cross-correlation is estimated. The longer
T is, the better the cross-correlation estimate E[C12(t
)]. For this function, there are 2 bearings or values of t
that can arise for the maximum value of E[C12(t
)]. One represents the back direction that we know is wrong because the
body of the torpedo blocks out that back direction. The other bearing
then has to be the correct one. Hydrophone separation, x, in homing torpedoes
is small but a relatively broad segment of the noise spectra is available
to provide sufficient bearing accuracy for tracking.
The cross-correlation function of Equation (1) cannot be realized in practice
but only estimated. Accuracy of the estimate depends primarily on 1) signal-to-noise
ratio (SNR), 2) bandwidth of the signal and 3) separation of the hydrophones.
Obviously for wider separations, bearing accuracy is better. The choice
of hydrophone separation is a compromise imposed by the operational requirements
arising when one wishes to place hydrophones on an ROV. Loss of coherence
depends on environmental conditions and their effect on propagation of
sound. Loss of coherence is more severe at the higher frequencies, but
it is not an important factor for arrays that will fit on an ROV. For
large signal bandwidths, the peak of the cross-correlation function is
narrow. When estimating the cross-correlation function, the time gate
T imposes a (sin pf)/pf type function upon the estimate that, along with
SNR, determines how accurately one can track a fish. When the bandwidth
is large and SNR high, one can choose a small time window and/or a small
hydrophone separation and get good homing results.
Methods
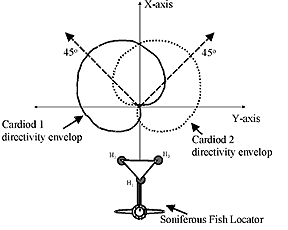 |
Figure 1. Illustration of the principle
of null steering on an acoustic source with two cardiod hydrophones.
The Soniferous Fish Locator consists of three hydrophones (H1-H3)
configured to form two orthogonal cardiods shown by the solid and
dotted lines. The two cardiods are 180 degrees out of phase with each
other. Summing the two results in a null along the x-axis. A bearing
to a sound source is obtained by rotating the SFL until the source
direction is coincident with the null. |
The SFL was designed to work based on the well-understood principle of
null steering on an acoustic source with two cardiod hydrophones (Fig.
1). Specifically, the SFL consists of three hydrophones configured to
form two orthogonal cardiods shown by the solid and dotted lines (Fig.
1). The two cardiods are 180 degrees out of phase with each other in this
configuration. Electronic summing of the two cardiods results in a null
along the x-axis (Fig. 1). A bearing to a sound source is obtained by
rotating the SFL until the sound direction is coincident with the null.
To enable an operator to determine bearing, output from the SFL will be
sent to both earphones and a recording device. The null is found by listening
to the summed output of the two cardiods in one ear while simultaneously
listening to the sound intensity with the other ear. Feeding output from
cardiod 1 prior to summation with cardiod 2 to the second earphone channel
eliminates noise from behind the SFL (sound from behind the SFL is nulled
out by the cardiod, Fig. 1). Hence, the operator listens only to the intensity
of sounds coming from in front of the SFL. This is an important property
of the SFL that reduces interference due to ROV noise and/or boat noise
when operating in shallow water. The distances, d, separating the hydrophones
can be changed to increase or decrease the sensitivity of the dipole and
allow the operator to tune the maximum sensitivity toward the predominant
frequency band of the type of soniferous fish species for which he/she
is searching. s
Initial tests of the feasibility of deploying an array of hydrophones
on a Phantom III model ROV as part of the SFL were conducted in test tanks
located at the Northeast-Great Lakes Center for the National Under Sea
Research Program at Avery Pt. Connecticut in October 2001. Test were conducted
on the array configuration, attachment methods and ROV noise production.
The ROV was not able to support a hydrophone array in the required configuration
(Fig. 1) because of ballast problems. We therefore had to modify the configuration
so that the hydrophone array could be supported by the ROV frame (Fig.
2). Unfortunately, this configuration does not allow for the cancellation
of ROV noise (the array must be forward of the noise source as in Fig.
1). With this configuration, noise levels under various operating conditions
were tested: 1) with all thrusters off and the ROV sitting on the bottom,
2) with top thrusters on, 3) with rear thrusters on, and 4) with all thrusters
on.
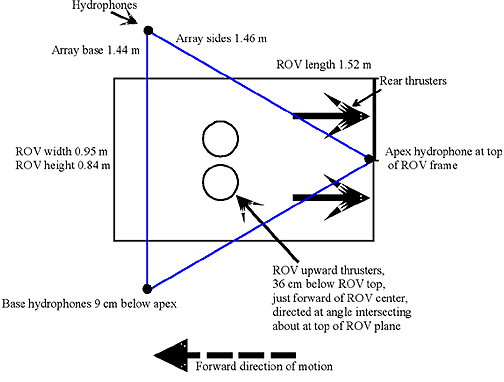 |
Figure 2. Schematic illustration of
the hydrophone array configuration and attachment to the Phantom III
ROV. |
Field testing was conducted within the Stellwagen National Marine Sanctuary
on board the R/V Connecticut from October 17-24, 2001. Ten ROV dives were
conducted in sand, gravel and boulder habitats within the sanctuary. Operations
were conducted in depths of up to 70 m under sometimes harsh environmental
conditions and strong tidal currents. To reduce ship noise, ROV dives
were conducted while the ship was at anchor and running off of its generators.
The array was composed of three TH608-40 model hydrophones made by Engineering
Acoustics, Inc (933 Lewis Drive, Suite C, Winter Park, FL 32789). The
hydrophones had a nominal sensitivity at the preamplifier output of 160.5
dB. The 3-channel audio data from the array was captured to a laptop PC
with a 4-channel I/O board and NIDisk software supplied by Engineering
Design (43 Newton St., Belmont, MA 02478). Sound signal processing was
conducted using Signal 4.0 (Engineering Design). A 1 k Hz sine wave was
played through a portable CD player into the system and the input voltage
recorded at the beginning of each ROV dive. This allowed calibration of
the system gain, in addition to the hydrophone. A single channel of audio
data was simultaneously recorded to video (both Hi-8 and VHS) for backup.
The calibration signal was also recorded to the videotape so that calibrated
audio data can be obtained directly from the tapes to obtain signal source
levels.
Results
Tank tests revealed a very high level of noise, even with the thrusters
turned off and the ROV sitting motionless on the bottom of the tank (Fig.
3). Although noise levels were highly variable, we estimated levels of
>130 dBV with thruster off and >160 dBV with all thrusters on. The
high level of noise precluded the operation of the SFL with a "flying"
ROV, with the current array configuration. We therefore decided to modify
the operation of the ROV while in the field in order to increase the signal
to noise ratio enough to obtain bearing information. We required the ROV
to remain stationary with its thrusters turned off long enough to acquire
the bearing to the sound source.
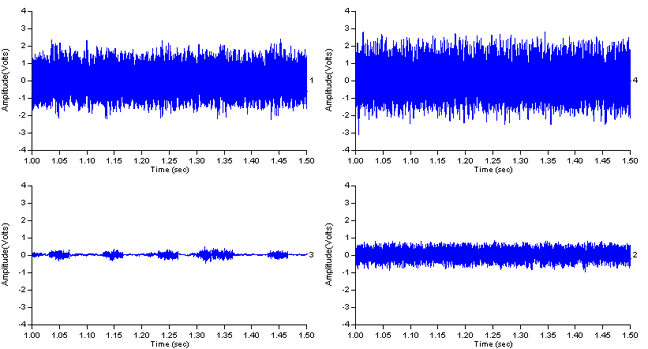 |
Figure 3. Tank test of noise generation
by the ROV with all thrusters off (lower left), top thrusters on (lower
right), back thrusters on (upper left) and all thrusters on (upper
right). Digitized at 20 k Hz. |
With all thrusters on, the ROV produced high levels of sound at both
high and low frequencies (Fig. 4). Dominant frequencies were centered
on 7-8 kHz. While the stationary ROV was significantly quieter, it still
generated substantial noise centered on 8 kHz (Fig. 5). The low frequency
noise in Fig. 5 is an artifact resulting from mechanical banging, rubbing
and tapping on the tank sides by technicians testing sound reception.
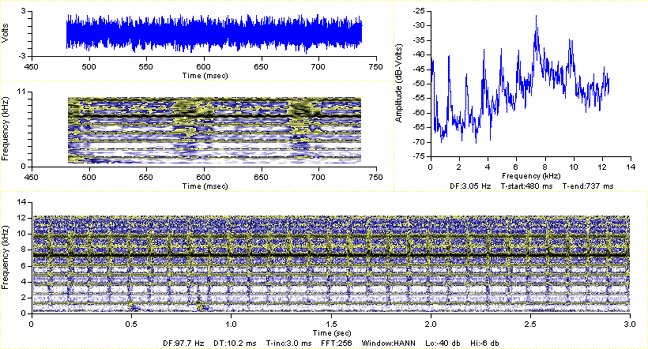 |
Figure 4. Noise generation from the
ROV with all thrusters on. Recorded at 20 kHz. |
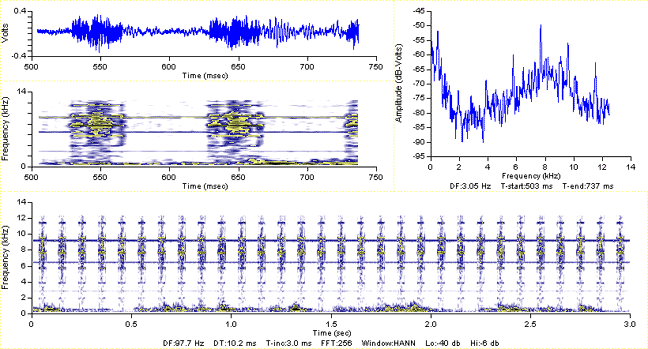 |
Figure 5. Noise generated by the ROV
while sitting on the tank bottom with all thrusters off. |
Recording fish sounds in the field with an array attached to the ROV
proved to be very difficult in practice. Strong currents limited our ability
to remain stationary on the bottom. The ROV was rarely able to maintain
its position on the bottom for more than a few minutes before the operator
was forced to turn on its thrusters to stabilize the vehicle. This also
required the operator to turn on the ROV lights, thus further disturbing
the fishes. Fish sounds were recorded on only one occasion when we were
able to maintain the ROV on the bottom with its thrusters and lights off
(Fig. 6). A prolonged series of low thumps and growls from a single fish
were recorded over a 20 minute period when the ROV was sitting stationary
with its lights off. During this time a large cusk, Brosme brosme, was
frequently observed hanging around the ROV. It is highly likely that the
cusk is the source of the recorded sounds. We estimated the ambient noise
(ROV + ship + seas) level at around 134 dBV and the cusk call at around
140 dBV. At other times when the lights and thrusters were on, cusk were
only observed in a highly agitated state, and appeared to strongly avoid
the ROV.
Discussion
Based on preliminary analysis of these data we feel that the concept of
a Soniferous Locator Device is viable. However, current ROV designs preclude
optimal configuration of the hydrophone array, requiring the SFL to be
operated in a stationary mode. We propose that a vehicle specifically
designed for low noise production and capable of carrying an SFL with
a 2-3 m base line in its nose would provide an exciting new passive acoustic
tool for soniferous fish surveys. The low calling rate of the fish recorded
in this study demonstrates that it would be difficult to track fish using
the manual null steering method proposed. Faster digital tracking using
this same principal would correct this problem and should be implemented
in future efforts. However, it is important to point out that data collected
during this cruise demonstrates that an ROV can serve as an adequate vehicle
for the collection of underwater acoustic data even without the SFL. The
ROV with a hydrophone attached would be used to locate an optimum location
and then would be set down on the bottom to record sounds. In this way,
a roving survey could be conducted.
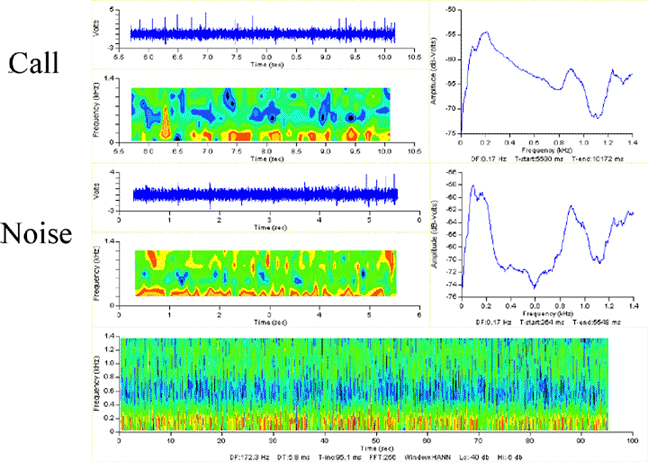 |
Figure 6. Recording of ROV/Ship and
ambient noise (bottom panel) together with the call of the cusk, Brosme
brosme. The spectrum of a 95 second sequence of multiple fish calls
of a single fish is shown in the bottom panel. The middle panels contain
relative amplitude waveform, spectra and power spectra for a 5 second
sequence containing only noise, while the upper panels contain a single
fish call (sampled at 20 kHz and filtered above 1400 Hz). |
Although cusk have long been considered to be soniferous because of the
presence of a sonic muscle, they had never been recorded until Norwegian
scientists recently recorded their spawning sounds (Aud Vold Soldal, Institute
of Marine Research, Norway, pers. Comm.). The calls apparently resemble
haddock spawning calls and are very different from those we recorded during
this study. Our recordings were conducted well outside of the spawning
season for cusk, so the sounds were likely associated with other behavior
(feeding or territorial display). Observations made subsequent to this
study revealed that cusk vigorously guard the chum bag attached to the
ROV and frequency chase away other fishes, suggesting the species is highly
aggressive and territorial. Because so little is known of the cusk's behavior,
ecology and habitat requirements, and because it appears to respond well
to a stationary ROV with its lights turned off, it makes a promising field
study animal for passive acoustic.
A secondary outcome of the cruise was that we obtained sufficient video
data to suggest that the behavior of some species is strongly influenced
by the ROV and/or the ROV lights. Adult cunner, redfish and pollock obviously
avoided the ROV during the day, but pollock were strongly attracted to
the ROV at night due to our use of chum and bright lights. The chum attracted
swarms of amphipods that in turn attracted a large aggregation of pollock
as well as haddock, cod and skates. Cusk were only observed in boulder
habitat and avoided the mobile ROV both during the day and night when
the lights were on. When only infrared lights were used, the cusk was
clearly attracted to the chum bag on the ROV and showed no avoidance of
a stationary ROV. Contrastingly, species such as cunner, redfish and silver
hake appear to avoid the ROV regardless of whether its lights are on or
off, or whether it is moving or stationary. The response of the cusk to
the mobile ROV with its lights turned on suggest the species strongly
avoids the ROV. It could not be determined whether the lights or the ROV
noise caused this avoidance, however subsequent observations of cusk behavior
indicate no avoidance of stationary cameras with white lights. We suggest
then, that the noise generated by the ROV can be a significant source
of bias in studies using ROVs for fish census.
Acknowledgements
We thank Meghan Hendry-Brogan for diligent work in both the field and
laboratory to collect and process fish sound data. Rebecca Jordan and
David Howe assisted in the field. This project received major funding
from the Northeast and Great Lakes National Undersea Research Center,
which also provided extensive logistical support. The Woods Hole Sea Grant
College Program also provided supporting funds. The Sounds Conservancy,
Quebec-Labrador Foundation/Atlantic Center for the Environment provided
a stipend for Megans fieldwork.
Literature Cited
Luczkovich, J.J., H.J. Daniel, III., M.W. Sprague, S.E. Johnson, R.C.
Pullinger, T. Jenkins, and M. Hutchinson. 1999. Characterization of critical
spawning habitats of weakfish, spotted seatrout and red drum in Pamlico
Sound using hydrophone surveys. Final Report and Annual Performance Report
Grant F-62-1 and F-62-2, Funded by the U.S. Department of the Interior,
Fish and Wildlife Service in Cooperation with the North Carolina Department
of Environment and Natural Resources, Division of Marine Fisheries, Morehead
City, NC 28557. 128 p.
Luczkovich, J.J., M.W. Sprague, S.E. Johnson, and R. C. Pullinger. 1999.
Delimiting spawning areas of weakfish Cynoscion regalis (Family Sciaenidae)
in Pamlico Sound, North Carolina using passive hydroacoustic surveys.
Bioacoustics 10:143-160.
Saucier, M.H., and D.M. Baltz. 1993. Spawning site selection by spotted
seatrout, Cynoscion nebulosus, and black drum, Pogonias cromis, in Louisiana.
Env. Biol. Fish. 36:257-272.
Return to Top | Workshop
Proceedings: Short Papers
|