New technologies
for passive acoustic detection of fish sound production
David A. Mann
University of South Florida, College of Marine Science, 140 Seventh
Avenue South, St. Petersburg, FL, 33701 USA. dmann@marine.usf.edu
Introduction
Passive acoustic detection of fish sounds relies heavily on advances
in recording and data processing technology. The recent explosion in
fast, inexpensive personal computers and electronics has created tremendous
growth potential in the field. This paper describes early efforts in
developing passive acoustic detection systems for fishes and more recent
efforts utilizing digital systems. The goals of each of these systems
were to automatically detect and quantify sounds of interest in real-time,
minimize false detections, and minimize the amount of data that needs
to be stored to determine calling rates continuously over long periods
of time.
First Generation Passive Acoustic Detection System
Most fish sounds are either simple pulsed broad-band sounds or tonal
type sounds, where the pulse rates or dominant frequency are species-specific
(e.g. Lobel and Mann, 1995; Mann and Lobel, 1998; reviewed in Zelick
et al., 1999). Fish sounds do not typically exhibit complex frequency
modulations seen in many marine mammal vocalizations. This makes it
possible to describe most fish sounds with a few metrics, such as sound
duration, peak frequency, and bandwidth. Timing between pulses can be
recorded by storing the time of onset of each pulse. By recording these
simple metrics, a system can be developed to automatically detect and
process sounds of interest and greatly reduce the amount of data that
would be acquired by simply recording continuously.
Early attempts at passive acoustic detection involved developing a largely
analog system that would detect sounds that were above some background
level and store the time of occurrence and sound duration (actual sound
data were not stored) (Mann and Lobel, 1995) (Fig. 1). From these data,
the rate of sound production of different species sounds could
be determined. This system was employed to measure sound production
rates of individual damselfish over periods of months, and revealed
a striking dawn chorus in sound production and a tight link between
sound production and spawning cycles (Mann and Lobel, 1995).
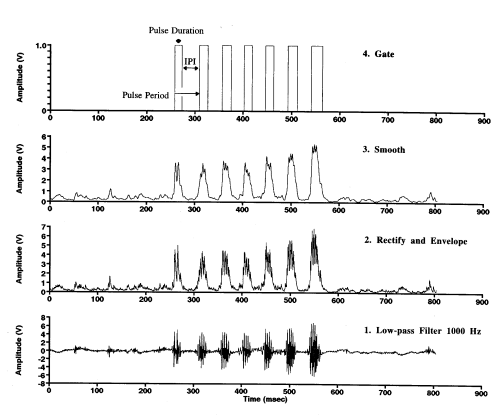 |
Figure 1.
Signal processing scheme for the detection of damselfish (Dascyllus
albisella) calls. |
Real-Time Digital
Signal Processing Systems
While the analog system was a robust detector, continued increases in
data storage capacities and the emergence of inexpensive digital signal
processing chips and the flexibility that these provide, prompted the
development of a programmable digital system. This system is commercially
produced by Tucker-Davis Technologies (Gainesville, FL) and consists
of a battery-powered datalogger with two channels of A/D, 32 MB of RAM,
and a graphical programming interface. The flexibility of the datalogger
is that it can be used to process the signal in real-time including
a wide array of filtering (FIR, IIR) techniques and adaptive thresholding.
The datalogger can be programmed to store whatever data is desired by
the researcher. To demonstrate its flexibility, a device was programmed
to detect the sounds produced by the toadfish Opsanus beta, store the
time of occurrence of the sound and record a 1000-point sound sample
(Fig 2).
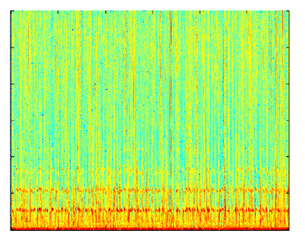 |
Figure 2.
Spectrogram of a series of automatically-detected toadfish (Opsanus
beta) calls plotted one after another. The dominant frequency of
these calls is approximately 250 Hz. |
The Future of
Fisheries Bioacoustics
The primary tools for the fish bioacoustician will remain the PC and
continuous digital recording systems for some time. To promote the emergence
of fisheries bioacoustics requires more research into the sounds made
by different fish species and the development of new technologies that
utilize these data.
Ultimately fisheries bioacoustics should move the way of fisheries acoustics
where the signal output is not the actual sound data, but the locations
and intensity of fish spawning. A useful analogy is the development
of SONAR systems for fish quantification. These systems do not deliver
raw sound data to the researcher. They return processed data on fish
location and abundance. One can envision the day when real-time fisheries
bioacoustics systems will produce maps of the locations of sound-producing
fishes that can provide managers with data on the temporal and spatial
extent of spawning.
Acknowledgments
My introduction and passion for the field of passive acoustic detection
was driven by my Ph.D. advisor Phillip Lobel. Support for the development
of the digital datalogger was provided to TDT by an NIH grant to the
author. I also thank Florida Sea Grant and the conference organizers
for supporting my participation.
References
Lobel, P.S. and D.A. Mann. 1995. Spawning sounds of the damselfish,
Dascyllus albisella (Pomacentridae), and relationship to male size.
Bioacoustics 6: 187-198.
Mann, D.A., and P.S. Lobel. 1995. Passive acoustic detection of sounds
produced by the damselfish, Dascyllus albisella (Pomacentridae). Bioacoustics
6: 199-213.
Mann, D.A., and P.S. Lobel. 1998. Acoustic behavior of the damselfish
Dascyllus albisella: behavioral and geographic variation. Environ. Biol.
Fish. 51: 421-428.
Zelick, R., D.A. Mann, and A.N. Popper. 1999. Acoustic communication
in fishes and frogs. In: Comparative Hearing: Fish and Amphibians. Springer,
New York. pp. 363-411.
Return
to Top | Workshop Proceedings: Short
Papers
|