EEL News
- Research Highlights
- 2015
- 2014
- 2013
- 2012
- 2011
- 2010
Previous EEL Research Highlights
Stoerzinger, K.A., W.T. Hong, E.J. Crumlin, H. Bluhm, M.D. Biegalski, and Y. Shao-Horn
The reactivity of water with the (001)pc surface of epitaxial LaCoO3 (LCO) thin films was investigated as a function of relative humidity (RH) by ambient pressure X-ray photoelectron spectroscopy. Significant changes were found in the O 1s and C 1s core-level spectra at different RHs, which were deconvoluted to yield new insights into the hydroxylation and hydration of the LCO surface. Continue reading.
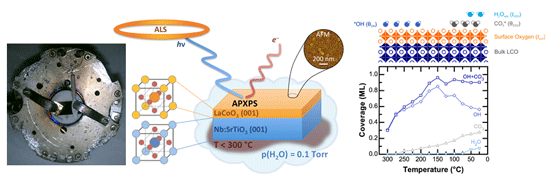
Zhenxing Feng, Yizhak Yacoby, Wesley Hong, Hua Zhou, Michael Biegalski, Hans Christen and Yang Shao-Horn
Surface segregation in metal oxides can greatly influence the oxygen transport and surface oxygen exchange kinetics critical to the performance of solid-state devices such as oxygen permeation membranes and solid oxide fuel/electrolytic cell electrodes. Unfortunately detecting elemental distributions at the atomic scale near the surface remains challenging, which hampers the understanding of underpinning mechanisms and control of surface segregation for the design of high-performance materials. Using the coherent Bragg rod analysis (COBRA) method, we report the first direct 3D atomic imaging of a 4 nm-thick “La0.8Sr0.2CoO3–δ”/SrTiO3 epitaxial film. Continue reading.
Researchers gained new insight into thin-film superiority by probing the structure of perovskites at the the U.S. Department of Energy's Advanced Photon Source (APS), Argonne National Laboratory. They used a groundbreaking approach to tease apart the thin-film structure and chemistry layer-by-layer. Continue reading.
Erika Gebel Berg, Argonne National Laboratory
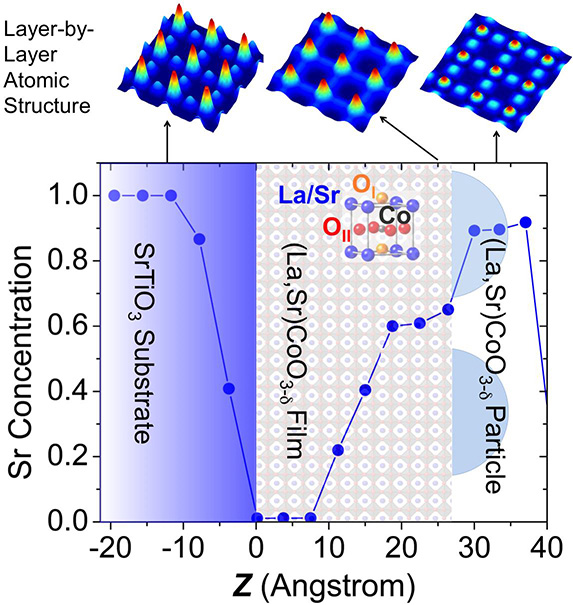
Alexis Grimaud, Kevin J. May, Christopher E. Carlton, Yueh-Lin Lee, Marcel Risch, Wesley T. Hong, Jigang Zhou and Yang Shao-Horn
New catalysts for the oxygen evolution reaction in basic solution are important for energy storage applications. Here, the authors report the high activity and stability of double perovskites in this role, and their performance is interpreted with regard to the proximity of the oxygen p-band to the Fermi level.
Watch a short movie of the catalyst evolving oxygen.
Highly active catalysts could be key to improved energy storage in fuel cells and advanced batteries.
David L. Chandler, MIT News Office
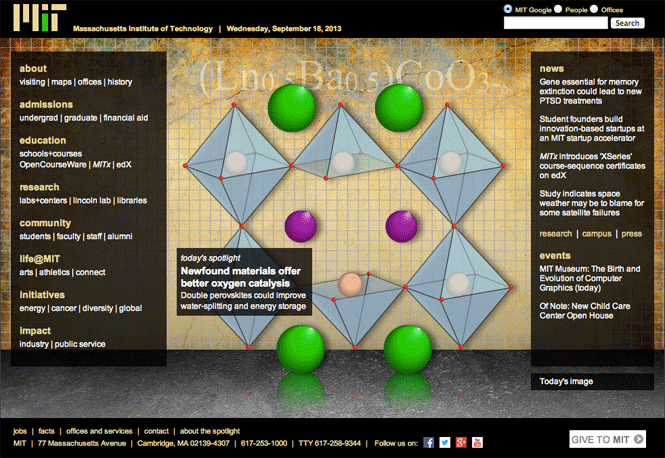
Li Zhong, Robert R. Mitchell, Yang Liu, Betar Gallant, Carl V. Thompson, Jian Yu Huang, Scott Mao and Yang Shao-Horn
In this Letter, we report the first in situ transmission electron microscopy observation of electrochemical oxidation of Li2O2, providing insights into the rate limiting processes that govern charge in Li–O2 cells. In these studies, oxidation of electrochemically formed Li2O2 particles, supported on multiwall carbon nanotutubes (MWCNTs), was found to occur preferentially at the MWCNT/Li2O2 interface, suggesting that electron transport in Li2O2 ultimately limits the oxidation kinetics at high rates or overpotentials.
Watch our movies of Li2O2 oxidation: movie 1, movie 2 and movie 3.
Imaging reveals what happens during charging; could lead to improved batteries for electric cars.
David L. Chandler, MIT News Office
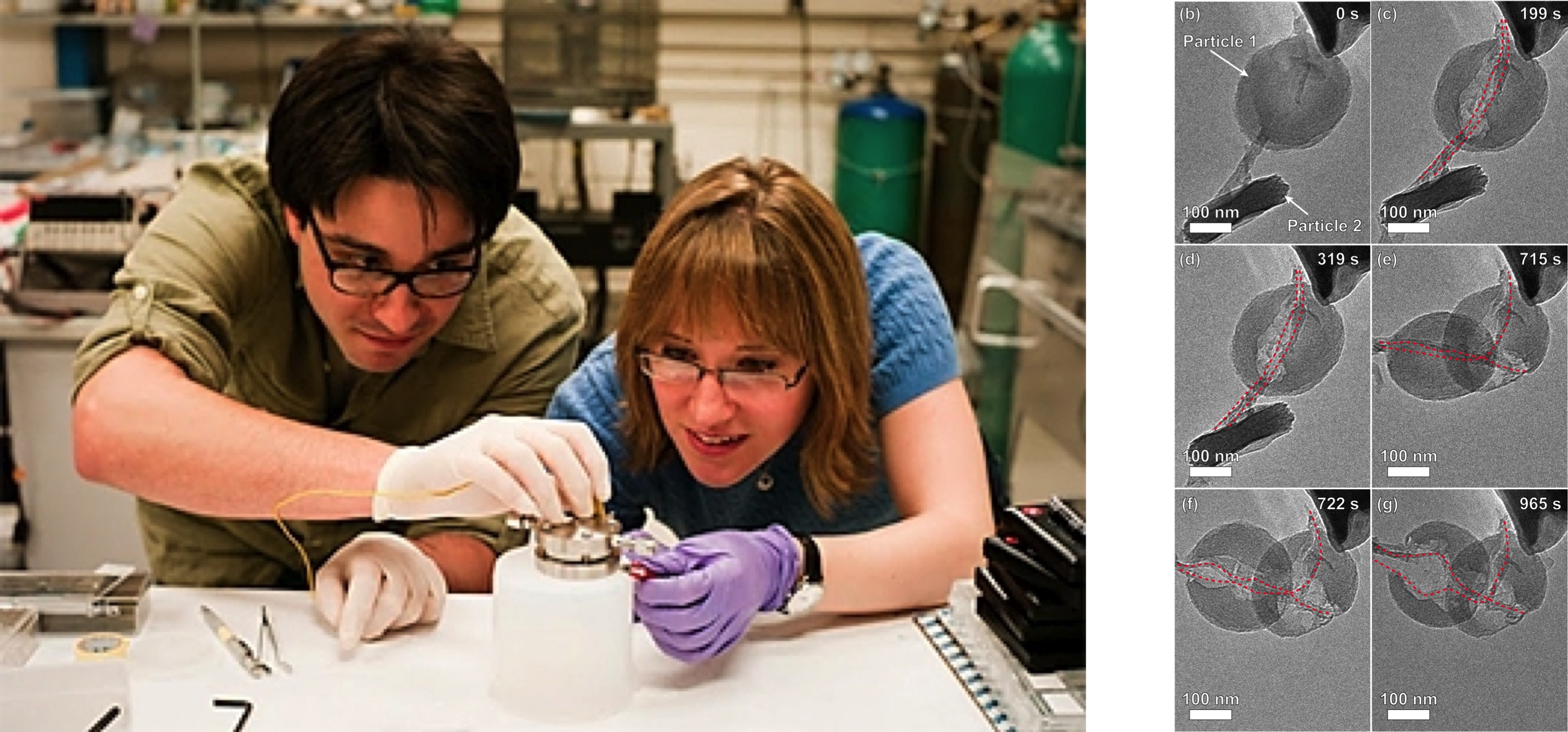
(Right) Oxidation of Li2O2 particles monitored by TEM.
Yi-Chun Lu, Ethan J. Crumlin, Gabriel M. Veith, Jonathon R. Harding, Eva Mutoro, Loïc Baggetto, Nancy J. Dudney, Zhi Liu & Yang Shao-Horn
The lack of fundamental understanding of the oxygen reduction and oxygen evolution in nonaqueous electrolytes significantly hinders the development of rechargeable lithium-air batteries. Here we employ a solid-state Li4+xTi5O12/LiPON/LixV2O5 cell and examine in situ the chemistry of Li-O2 reaction products on LixV2O5 as a function of applied voltage under ultra high vacuum (UHV) and at 500 mtorr of oxygen pressure using ambient pressure X-ray photoelectron spectroscopy (APXPS). Under UHV, lithium intercalated into LixV2O5 while molecular oxygen was reduced to form lithium peroxide on LixV2O5 in the presence of oxygen upon discharge. Interestingly, the oxidation of Li2O2 began at much lower overpotentials (~240 mV) than the charge overpotentials of conventional Li-O2 cells with aprotic electrolytes (~1000 mV). Our study provides the first evidence of reversible lithium peroxide formation and decomposition in situ on an oxide surface using a solid-state cell, and new insights into the reaction mechanism of Li-O2 chemistry. more
Fundamental reactions behind advanced battery technology, revealed in detail by advanced imaging method, could lead to improved materials.David L. Chandler, MIT News Office
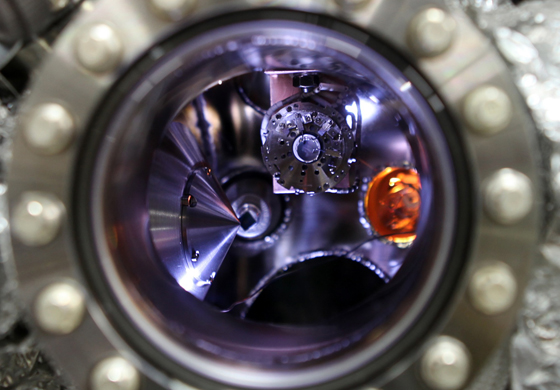