Thermal Materials
Despite the fact that heat is everywhere and we need to transfer heat from one place to another all the time, nature has not given us many good choices of materials to handle this job. The thermal conductivity of materials spans only five orders of magnitude (excluding vacuum): from the low end of air at 0.026 W/m-K to the high end of diamond ~2000 W/m-K at room temperature. In comparison, electrical conductivity spans over 30 orders of magnitude (excluding superconductivity). For some applications, we need better thermal insulators, while for others, we want to have better heat conductors. We have been working on a variety of materials and structures to meet the task of different applications, combining first principles simulations, modeling with experiments.
High Thermal Conductivity Semiconductors and Dielectrics
The most thermally conductive 3D material is diamond (k~2000 W/m-K). The next to come to mind is often copper around 400 W/m-K, significantly lower than diamond. In 2018, we and our collaborators demonstrated that a new material, boron arsenide (BAs), has a thermal conductivity around 1200 W/m-K at room temperature, making it the second best heat conductor after diamond. In 2019, we demonstrated that isotopically enriched cubic boron nitride (cBN) has a thermal conductivity around 1600 W/m-K at room temperature. Thus, BAs and cBN are now among the best heat known conductors. Furthermore, we predicted via first principles simulations that BAs also has very good electron mobility. With a bandgap of ~2 eV, BAs is a semiconductor with excellent potential. We are now working with our materials collaborators to grow BAs and measure its electrical and thermal properties.
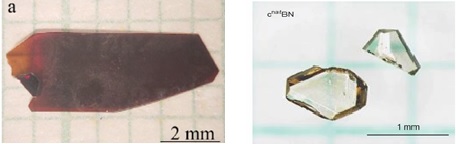
Key Publications
- Ke Chen, Bai Song, Navaneetha K. Ravichandran, Qiye Zheng, Xi Chen, Hwijong Lee, Haoran Sun, Sheng Li, Geethal Amila Gamage, Fei Tian, Zhiwei Ding, Qichen Song, Akash Rai, Hanlin Wu, Pawan Koirala, Aaron J. Schmidt, Kenji Watanabe, Bing Lv, Zhifeng Ren, Li Shi, David G. Cahill, Takashi Taniguchi, David Broido, and Gang Chen, “Ultrahigh thermal conductivity in isotope-enriched cubic boron nitride,” Science, 367, 555-559, 2020.
- Fei Tian, Bai Song, Xi Chen, Navaneetha K. Ravichandran4, Yinchuan Lv, Ke Chen, Sean Sullivan, Jaehyun Kim, Yuanyuan Zhou, Te-Huan Liu, Miguel Goni, Zhiwei Ding, Jingying Sun, Geethal Amila Gamage Udalamatta Gamage, Haoran Sun, Hamid Ziyaee, Shuyuan Huyan1, Liangzi Deng, Jianshi Zhou, Aaron J. Schmidt, Shuo Chen, Ching-Wu Chu, Pinshane Y. Huang, David Broido, Li Shi, Gang Chen, Zhifeng Ren, “Unusual High Thermal Conductivity in Boron Arsenide Bulk Crystals”, Science, 361, 582-585, 2018.
- Te-Huan Liu, Bai Song, Laureen Meroueh, Zhiwei Ding, Qichen Song, Jiawei Zhou, Mingda Li, and Gang Chen, “Simultaneously high electron and hole mobilities in cubic boron-V compounds (BP, BAs and BSb),” Physical Review B Rapid Communications, 98, 081203, 2018.
- Keivan Esfarjani, Gang Chen, and Harold T. Stokes, “Heat Transport in Silicon from First Principle Calculations,” Physical Review B, 84, 085204, 2011.
Thermoelectric Materials
Thermoelectric materials can convert heat directly into electrical energy by exploiting the thermal energy carried by charges (electrons and holes). Thermoelectric materials can be used for power generation as well as solid state refrigeration. The efficiency of thermoelectric power generators is determined by the nondimensional figure-of-merit, ZT=S2σT/k, where S is the Seebeck coefficient, σ the electrical conductivity, k the thermal conductivity, and T the absolute temperature. Over the past two decades, we have exploited different nanoscale physics to improve materials’ ZT, especially reduced thermal conductivity via boundary- and interface-scattering phonons. We have collaborated with material specialists, especially Prof. Zhifeng Ren at the University of Houston and the late MIT Institute Professor Mildred S. Dresselhaus, to advance the field of thermoelectrics. The nanocomposite approach we reported is widely explored by many groups around the world. We have developed strong simulation and experimental capabilities via these efforts and invented new devices and systems. Commercialization of the nanostructured thermoelectric materials was attempted via a startup for potential applications in vehicle water recovery and combined heat and electricity in solar thermal systems and home boilers.
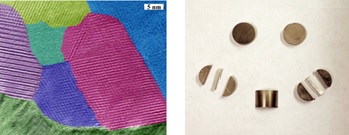
Key Publications
- Jun Mao, Hangtian Zhu, Zhiwei Ding, Zihang Liu, Geethal Amila Gamage, Gang Chen, and Zhifeng Ren, “High Thermoelectric Cooling Performance of n-type Mg3Bi2-Based Materials,” Science, 365, 495-498, 2019.
- Jiawei Zhou, Hangtian Zhu, Te-Huan Liu, Qichen Song, Ran He, Jun Mao, Bolin Liao, David Singh, Zhifeng Ren, Gang Chen, “Large thermoelectric power factor from crystal symmetry-protected non-bonding orbital,” Nature Communications, 9, 1721, 2018.
- M. Zebarjadi, K. Esfarjani, M.S. Dresselhaus, Z.F. Ren, and G. Chen, “Perspectives on Thermoelectrics : from fundamentals to device applications,” Energy and Environmental Science, Vol. 5, pp. 5147-5162, 2012.
- Bed Poudel, Qing Hao, Yi Ma, Yucheng Lan, Austin Minnich, Bo Yu, Xiao Yan, Dezhi Wang, Andrew Muto, Daryoosh Vashaee, Xiaoyuan Chen, Junming Liu, Mildred S. Dresselhaus, Gang Chen, and Zhifeng Ren, “High Thermoelectric Performance of Nanostructured Bismuth Antimony Telluride Bulk Alloys,” Science, 320, 634, 2008.
- M.S. Dresselhaus, G. Chen, M.Y. Tang, R. Yang, H. Lee, D.Z. Wang, Z.F. Ren, J.P. Fleurial, and P. Gogna, “New Directions for Thermoelectric Materials,” Advanced Materials, 19, 1-12, 2007.
High Thermal Conductivity Polymers
It is well known that polymers are poor heat conductors. Most polymers have a thermal conductivity <0.5 W/m-K. However, we believe that polymers can be engineered into good thermal conductors. Taking polyethylene as an example, it has carbon-carbon bonds along its molecular chain, yet the best heat conductor diamond is based on carbon-carbon bonds! In fact, inspired by the work of Fermi and his collaborators, we predicted that a single polyethylene molecule can have infinite thermal conductivity. This has not been proven experimentally yet. We have moved on to stretch polyethylene into nanofibers and plastic sheets and have achieved over 100 W/m-K in polyethylene nanofiber and over 60 W/m-K in polyethylene sheets along the stretching direction, making them comparable to or better than most metals in their thermal conductivity values. We also demonstrated an order of magnitude improvement in polymer thin films obtained via chemical vapor deposition. In general, we believe that there is plenty of room to engineer polymers into good heat conductors and are pursuing research in this direction via combined simulations, materials synthesis, and measurements.
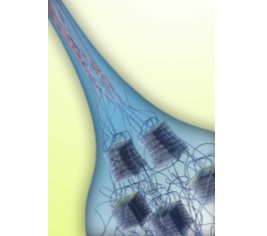
Key Publications
- Yanfei Xu, Daniel Kraemer, Bai Song, Zhang Jiang, Jiawei Zhou, James Loomis, Jianjian Wang, Mingda Li, Hadi Ghasemi, Xiaopeng Huang, Xiaobo Li and Gang Chen, “Plastic Films with High Thermal Conductivity” Nature Communications, 10, 1711, 2019.
- Yanfei Xu, Xiaoxue Wang, Jiawei Zhou, Bai Song, Jiang Zhang, Elizabeth M. Y Lee, Sam Huberman, Karen K. Gleason, and Gang Chen, “Molecular engineered conjugated polymer with high thermal conductivity,” Science Advances, eear3031, 2018.
- Sheng Shen, Asegun Henry, Jonathan Tong, Ruiting Zheng, and Gang Chen, “High Thermal Conductivity Polyethylene Nanofibers,” Nature Nanotechnology, 5, 251-255, 2010.
- A. Henry and G. Chen, “High Thermal Conductivity of Single Polyethylene Chains Using Molecular Dynamics Simulations,” Physical Review Letters, 101, 235502, 2008.
Hydrogels
Hydrogels, such as Jell-O, are water-polymer mixtures in quasi-solid form. There has been lots of exciting work in hydrogels for their biomedical applications. Our interests in hydrogel stem from our work on polymer heat conductors and floating solar absorbers for steam evaporation, being further stimulated by reports from others that hydrogels can be leveraged to achieve a much lower “apparent” latent heat of water and as moisture adsorbents. We are now working on understanding heat conduction mechanisms, thermodynamics, and vapor and ion transport in hydrogels.
Fabrics and Photon Engineered Structures
Fabrics are an important part of a daily life and regulate our body energy exchange with the environment. While vapor transport, conduction, and convection mechanisms are explored extensively in the making of fabrics, thermal radiation is another channel that remains to be explored. We invented an approach to make visible-opaque, infrared-transparent fabrics by exploring the different scattering regimes of fabrics to visible and infrared light. If more body heat can escape through the fabrics, we can dial up our air conditioning temperature and reduce energy consumption in buildings. In addition to fabrics, we are also developing other materials to engineer photon transport. In collaboration with Prof. Evelyn Wang’s group, we developed optically-transparent and infrared-opaque aerogels for solar thermal applications and window retrofitting. We are also working on thermally-regulated roofs and low-cost window retrofittings.
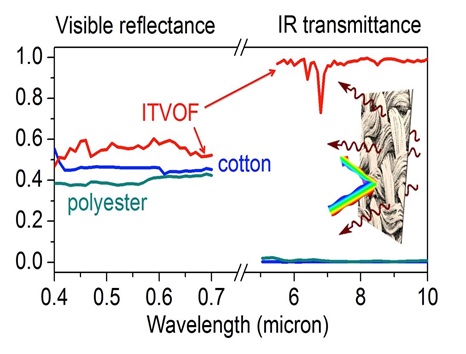
Key Publications
- Luis Marcelo Lozano, Seonggdon Hong, Yi Huang, Hadi Zandavi, Yassine Ait El Aoud, Yoichiro Tsurimaki, Jiawei Zhou, Yanfei Xu, Ricahrd M. Osgood III, Gang Chen, and Svetlana V. Boriskina, “Optical engineering of polymer materials and composites for simultaneous color and thermal management,” Optical Materials Express, 9, 1990-2005, 2019.
- Jonathan K. Tong, Xiaopeng Huang, Svetlana V. Boriskina, James Loomis, Yanfei Xu, and Gang Chen, “Infrared-Transparent Visible-Opaque Fabrics for Wearable Personal Thermal Management,” ACS Photonics, 2, 769-778, 2015.
Nano and Microfluids
Around 2000, researchers observed that some colloids with nanosized particles suspended in liquids have significantly enhanced thermal conductivity that could be explained using effective media theories. We were asked by an industrial sponsor to understand the mechanisms underpinning this phenomenon. Via some innovative experiments, we eventually understood that one major mechanism is the clustering of the nanoparticles and the percolation path the particles form. With this understanding, we decided that the best way to increase thermal conductivity is actually using micron sized thin sheets, such as graphite flakes. We also observed interesting thermal percolation phenomena. Freezing such fluids also leads to the ability to switch thermal and electrical conductivity.

Key Publications
- Lei Ma, Jianjian Wang, Amy Marconnet, Gareth McKinley, Wei Liu, Gang Chen, “Viscosity and Thermal Conductivity of Graphite Suspensions Near Percolation,” Nano Letters, 15, 127-133, 2015.
- J. J. Wang, R. T. Zheng, J. W. Gao, and G. Chen “Heat Conduction Mechanisms in Nanofluids and Suspensions,” Nano Today, 7, 124-136, 2012.
- R.T. Zheng, J.W. Gao, J.J. Wang, H.P. Feng, H. Ohtani, Jinbo Wang, and G. Chen, “Thermal Percolation in Stable Graphite Suspensions” Nano Letters, 12, 188-192, 2012.
- Ruiting Zheng, Jinwei Gao, Jianjian Wang, and Gang Chen, “Reversible temperature regulation of electrical and thermal conductivity using liquid-solid phase transitions,” Nature Communications, 2, 289, 2011.
- J.W. Gao, R.T. Zheng, H. Ohtani, D.S. Zhu, and G. Chen, “Experimental Investigation of Heat Conduction Mechanisms in Nanofluids--- Clue on Clustering,” Nano Letters, 9, 4128-4132, 2009.