|
The central theme of my research has been focused on the investigation of mass and heat transport processes in electrochemical systems including polymer electrolyte membrane fuel cells (PEFCs), all-vanadium redox flow batteries (VRFBs), and electrochemical sensors (ESs). Funding for these projects have been provided by the Department of Energy, Office of Naval Research, Volkswagen, and other government agencies, as well as companies (e.g. W. L. Gore and Associates).
In brief, the major highlights of my research can be summorized as:
All-Vanadium Redox Flow Batteries |
(1) Investigated the vanadium ion and water transport through polymeric membranes for all-vanadium redox flow batteries (VRFBs) via design, engineering and prototyping a novel experimental approach (equipped with UV/Vis spectroscopy) along with developing mathematical models that include interactions between the contributing ionic species present in VRFBs.
|
(2) Invented a novel cell design for passive mitigation of capacity decay and crossover in redox flow batteries.
|
(3) Developed a passive approach utilizing asymmetric negative and positive electrolyte composition for mitigating radical capacity decay in redox flow batteries.
|
Polymer Electrolyte Membrane Fuel Cells (PEMFCs) |
(4) Demonstrated a passive approach for mitigating liquid water flooding in cathode electrodes using asymmetric heat and mass transport properties of gas diffusion layers in polymer electrolyte fuel cell (PEFCs).
|
Electrochemical Sensors (ESs) |
(5) Invented a novel cell design for passive mitigation of capacity decay in flow batteries.
|
My experimental and modeling experiences in various electrochemical systems have developed my expertise in phenomena related to electrochemistry, fluid mass and heat transport and transport in membranes.
My interests are in the high-performance Electrochemical Energy Storage and Conversion Devices (EESCDs), Thermal Storage Devices (TSDs), Solar Thermophotovoltaics (STPVs) and Water Desalination.
1. Polymer Electrolyte Membrane Fuel Cells (PEFCs):
Water transport across the PEFCs occurs by several driving modes, including diffusion, pressure gradient (gas phase or capillary pressure differential), electro-osmosis drag, thermos-osmosis and phase-change-induced flow. Therefore, water management remains a challenging topic in PEFCs. The membrane’s transport properties are a strong function of water content within the membrane. Extensive dry-out at the anode side and subsequent conductivity loss results in poor overall performance. For PEFC systems operating at high current densities such as those found in automotive applications, anode dry-out resulting from excessive electro-osmotic flux of water to the cathode has been shown to be a key limiting phenomenon.
a) Engineering water transport across the ion-exchange membrane in PEFCs using asymmetric MPL configuration
Water transport across the PEM has been engineered using asymmetric mass and heat transport properties of the micro-porous layer (MPLs) and the effect of asymmetric configuraiton has been investigated on the net water flux coefficient for extremes of wet and dry operating conditions via conducting extensive experimental tests. It is shown that with an asymmetric configuration, the net flux of water can be reversed under certain conditions, greatly enhancing high current density performance.
|
Engineerng the water transport across the PEFC
|
|
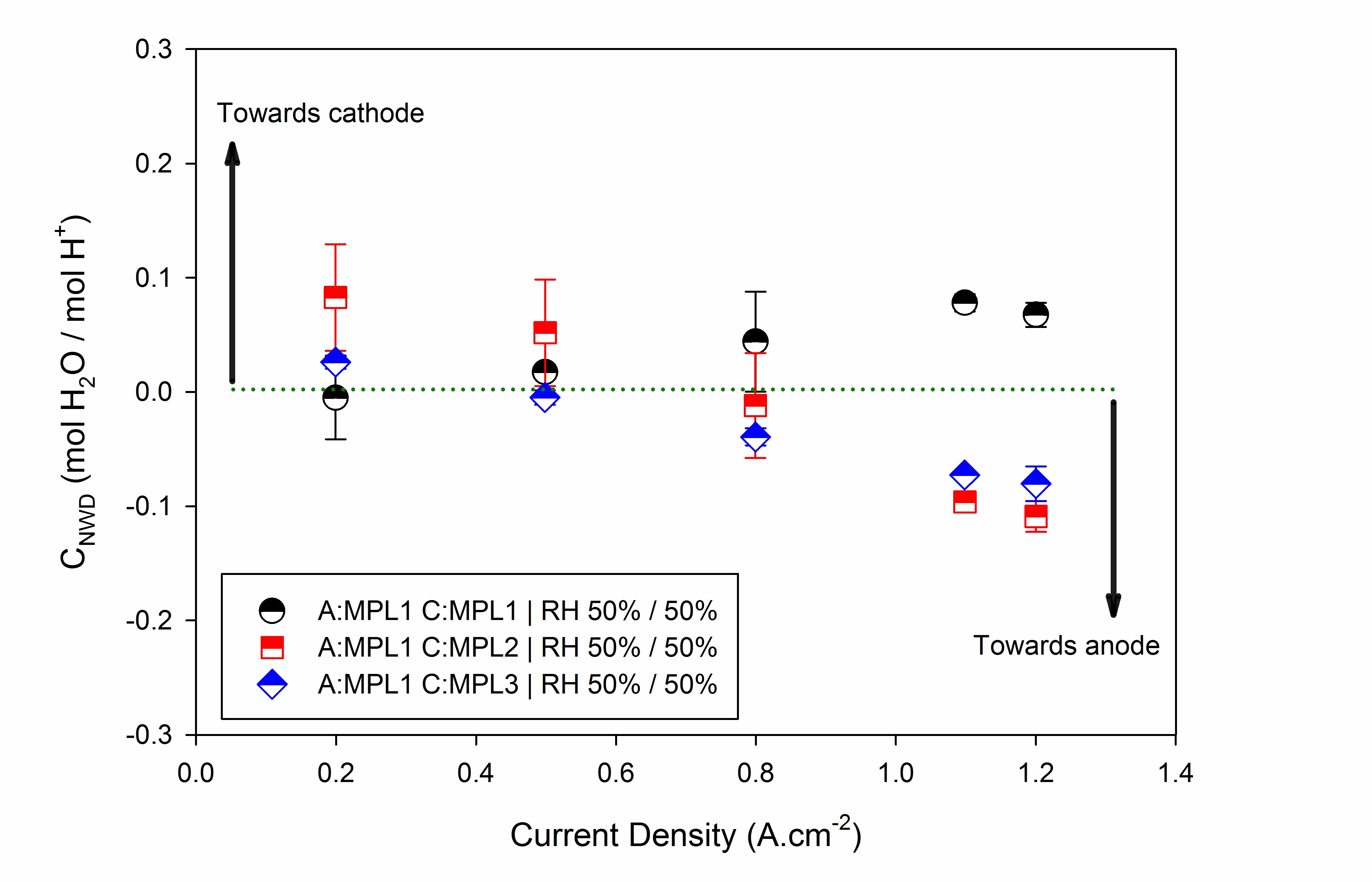
Real-time net water drag coefficient measurement along with polarization curves were used to engineer the back flux of water in PEFCs
|
b) A mathematical model for investigating the effect of temperature gradient on the net drag coefficient
A mathematical model has been developed to investigate the effect of temperature gradient on the net water drag coefficient.
|
Mathematical model for predicitng the temperature distribution across PEFC
|
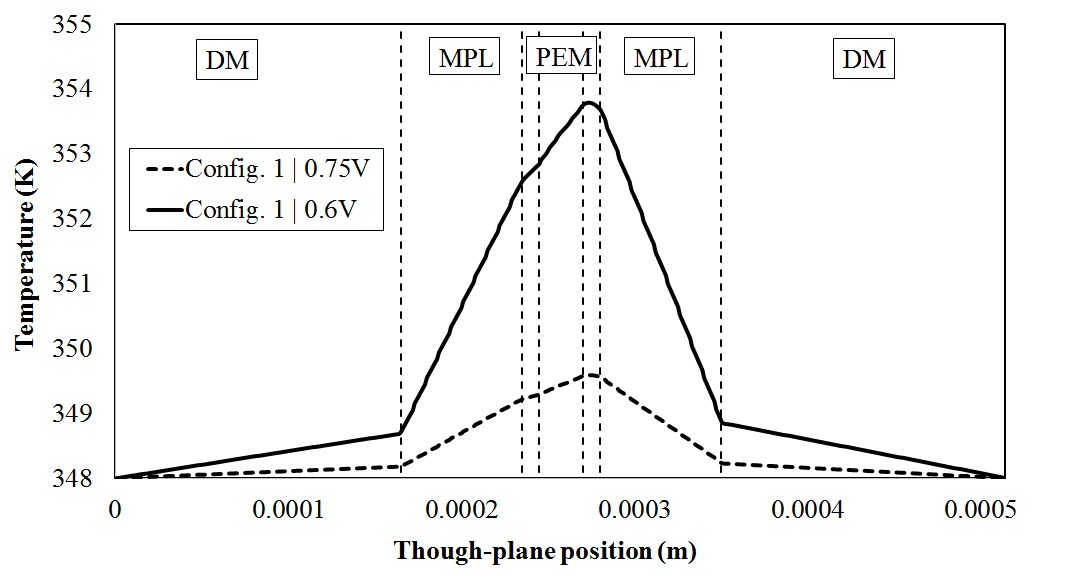
Predicted temperature distribution in through plane direction in an operating PEFC |
Related papers:
- Yasser Ashraf Gandomi, M. D. Edmundson, F. C. Busby, M. M. Mench, “Water Management in Polymer Electrolyte Fuel Cells through Asymmetric Thermal and Mass Transport Engineering of the Micro-Porous Layers”, Journal of Electrochemical Society, 163(8), F933-F944 (2016).
- Yasser Ashraf Gandomi, M. M. Mench, “Assessing the Limits of Water Management using Asymmetric Micro-Porous Layer Configurations”, ECS Transactions, 58(1), 1375-1382 (2013).
back to top
2. All-Vanadium Redox Flow Batteries (VRFBs):
Redox flow batteries (RFBs) are one of the most promisingtechnologies for grid scale energy storage systems and can be either aqueous or non-aqueous, based on their electrolytes.
Different RFB chemistries have been proposed; among the aqueous flow batteries, the all-vanadium, iron-chromium and hydrogen-bromine redox flow batteries are the most extensively studied. All-vanadium redox flow batteries (VRFBs) are unique among other redox flow batteries since they utilize the same ion with different oxidation states at both anode and cathode; they do not suffer from cross contamination, although crossover does result in selfdischarge
a) A novel system designed for measuring the crossover of vanadium ions and water in real-time
One of the major sources of capacity loss in all-vanadium redox flow batteries is the undesired transport of active vanadium species across the ion-exchange membrane, generically termed crossover. The concentration gradient and the electric field (via migration and electro-osmosis) are the main driving forces for vanadium ions transport across the membrane. Water transport across the ion-exchange membrane is due to multiple driving forces including the electro-osmotic drag, thermo-osmosis, osmotic and hydraulic pressure gradient.
In this work, a novel system (we call the set-up CSTTP (concentrated solution theory test protocol)) including multiple cells has been designed and built to investigate the crossover of vanadium species and water transport through the membrane in real-time. The test system utilizes ultraviolet/visible (UV/Vis) spectroscopy and is capable of separating contributions to crossover stemming from concentration and electrostatic potential gradients.
It is shown that the rate of species transport through the ion-exchange membrane is state of charge dependent and, as a result, interaction coefficients have been deduced which can be used to better estimate expected crossover over a range of operating conditions.
|
Schematic of CSTTP set-up (CSTTP enables measuring the crossover of vanadium ions and water in real-time) |
|
|
|
Permeability values were measured for vanadium ions and it was shown that the permeability values are state of charge dependent and accordingly, interaction coefficients deduced |
The electric field was shown to increase the negative-to-positive transport of V(II)/V(III) and suppress the positive-to-negative transport of V(IV)/V(V) during discharge, with an inverse trend during charging conditions. Electric-field induced transport coefficients were deduced directly from experimental data.
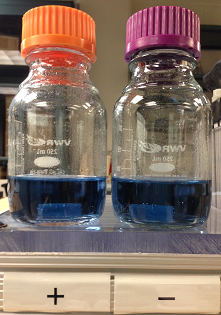
Crossover of V(II)/V(III) due to concentration gradient (symmetric crossover)
|
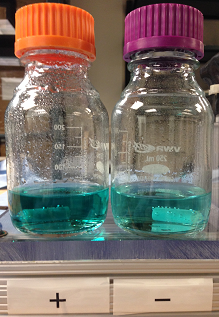
Crossover of V(IV)/V(V) due to concentration gradient (symmetric crossover)
|
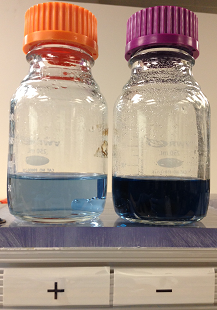
Crossover of V(II)/V(III) due to concentration gradient and electric field (asymmetric crossover) |
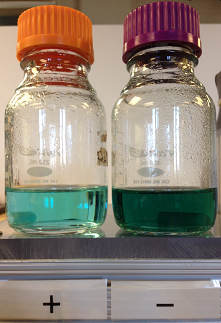
Crossover of V(IV)/V(V) due to concentration gradient and electric field (asymmetric crossover) |
b) Concentrated Solution Model of Transport in All Vanadium Redox Flow Battery Membrane Separator
A model of transport across the ion-exchange membrane in allvanadium redox flow batteries has been proposed based on concentrated solution theory for species with high concentration.
The model is based upon the Stefan-Maxwell multicomponent
diffusion equation where the fluxes of the species including
protons, bisulfate, water and the sulfonate
functional groups are fully coupled. The driving force for
species transport has been modeled in terms of concentration and electrostatic potential gradients. The ionic transference numbers as well as water electro-osmosis drag coefficient has been calculated for different acid concentrations.
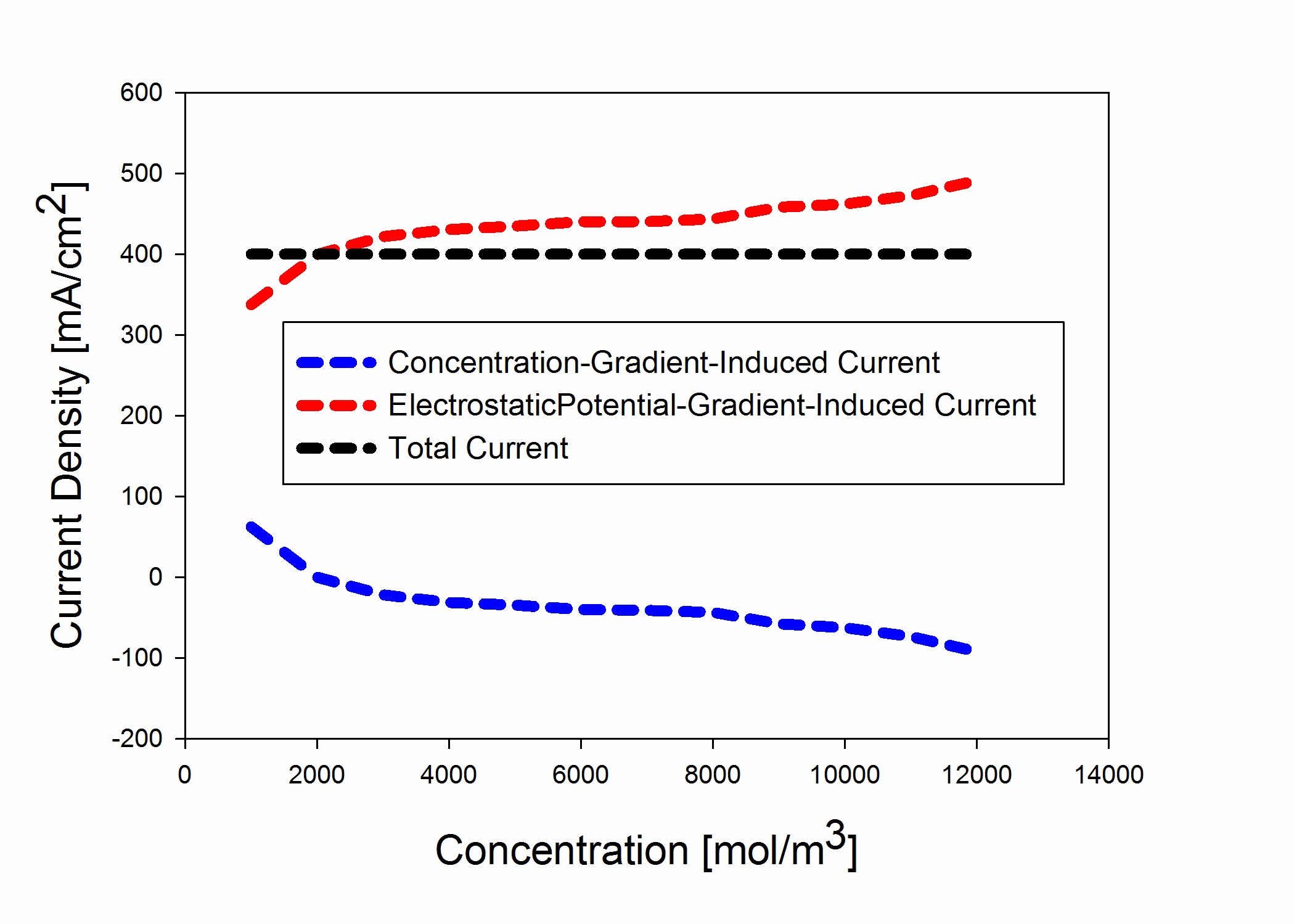
Concentrated solution theory was used for modeling the contribution to the overall current stemming from concentration gradient and electrostatic potential gradient
|
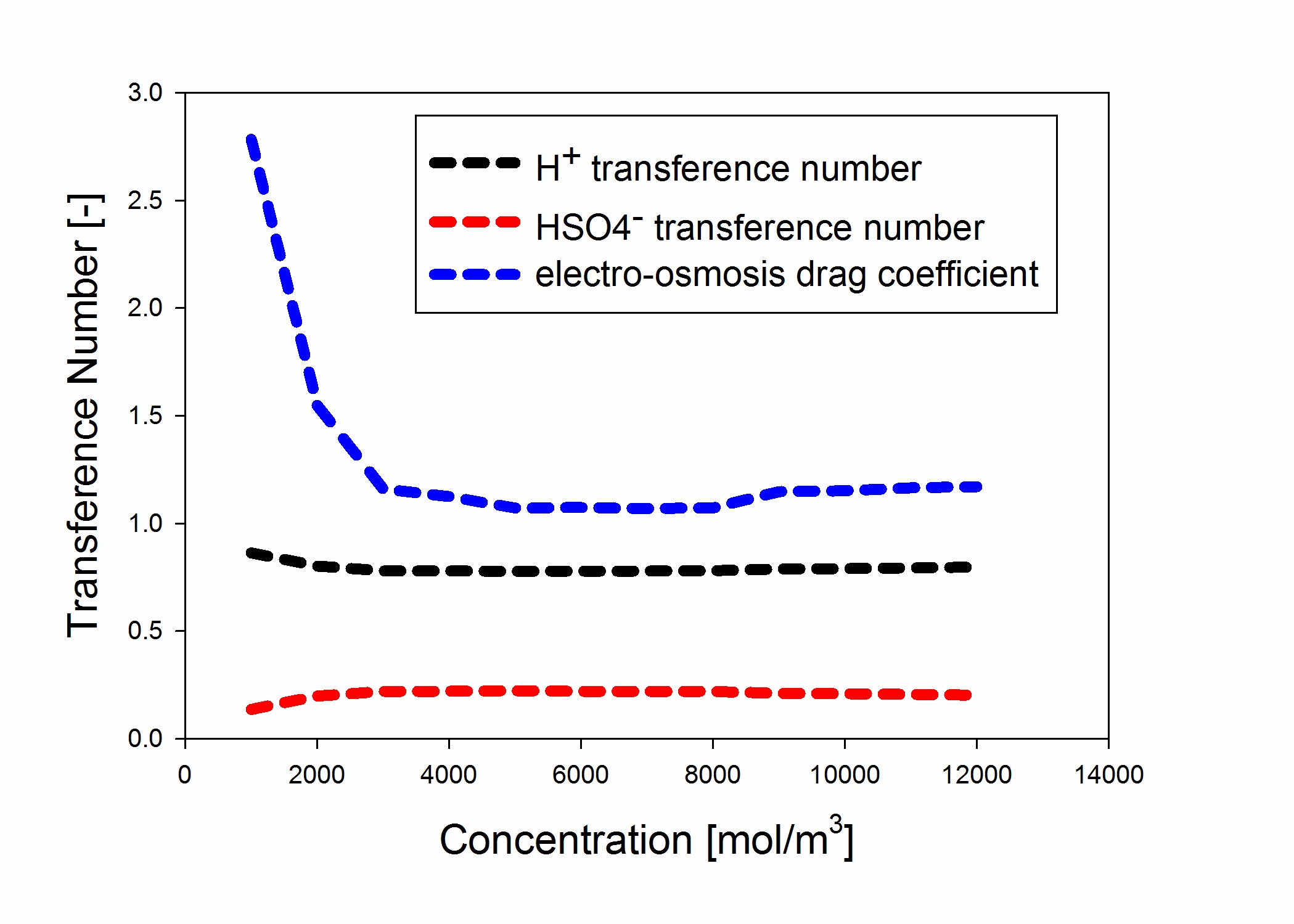
Predicting ionic transference numbers along with electro-osmotic drag coefficient of water using a model developed based on concentrated solution theory
|
c) Real-time in situ potential distribution measurement in all-vandium redox flow batteries
In this work, a diagnostic technique is established based on the implementation of a dynamic hydrogen electrode (DHE) for in situ measurement of the local potential distribution within the multilayer electrodes of the VRFB.
Micro-scale potential probes enabled in situ measurement of
local potential in electrode layers between the cell flow field and membrane. The local redox potentials were recorded for different
operating conditions and states of charges.
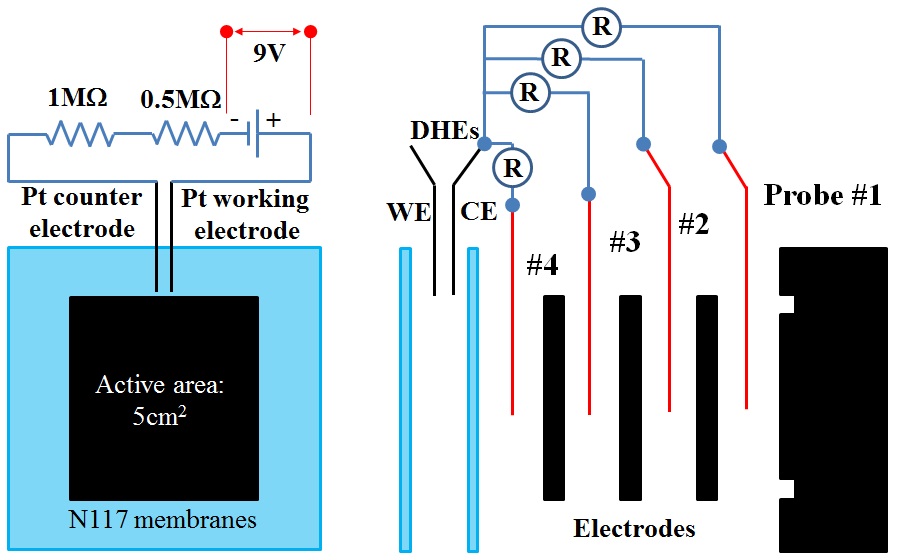
Potential distribution diagnostics for VRFBs
|
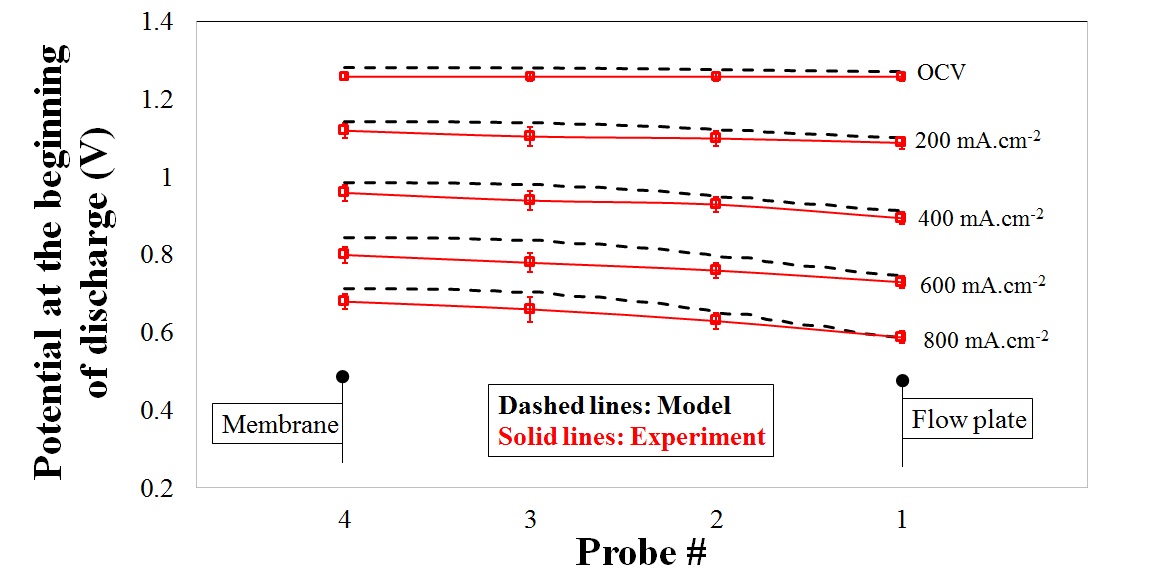
The potential distribution diagnostics was used to record the local redox potentials for different operating conditions and state of charges for an operando VRFB
|
d) A mathematical model was developed to predict the performacne, ionic species distributins, and potential distribution for an operando VRFB
To further analyze the behavior of potential distribution in the through-plane direction, a mathematical model was developed and the species distribution as well as the flux density of any individual component was modeled in terms of contributions from convective, diffusive and electrophoretic fluxes at each operating condition.
Both mathematical simulation and experimental data confirmed the distribution of potential in the through plane direction as a function of discharge current density, predicting the lowest potential in a region close to the flow plate.
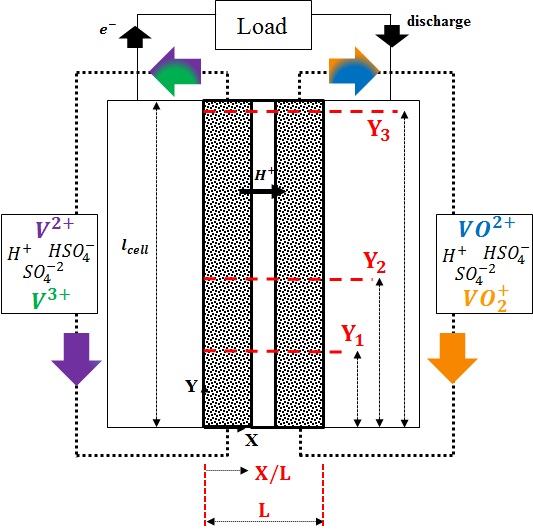
Computational domain for simulating the performance of a VRFB
|
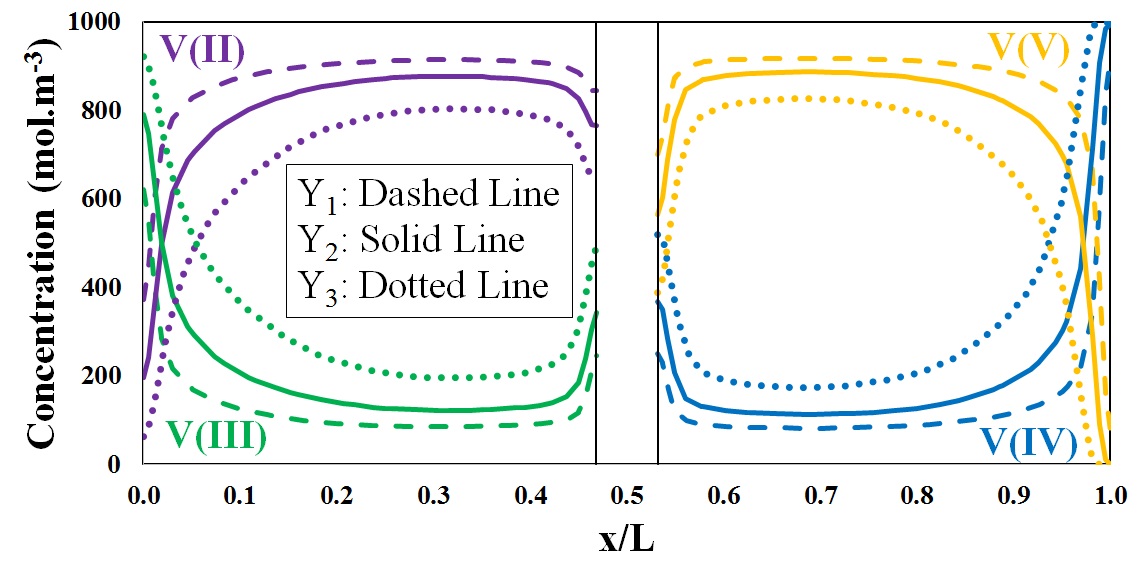
Vanadium ions concentration distribution
|
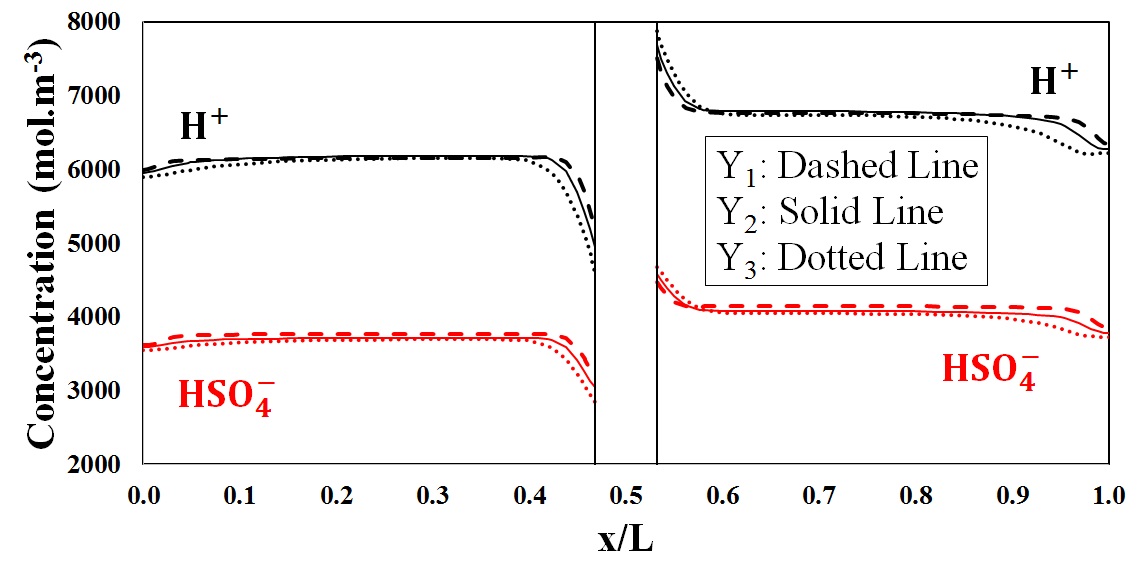
Proton and bisulphate concentration distribution
|
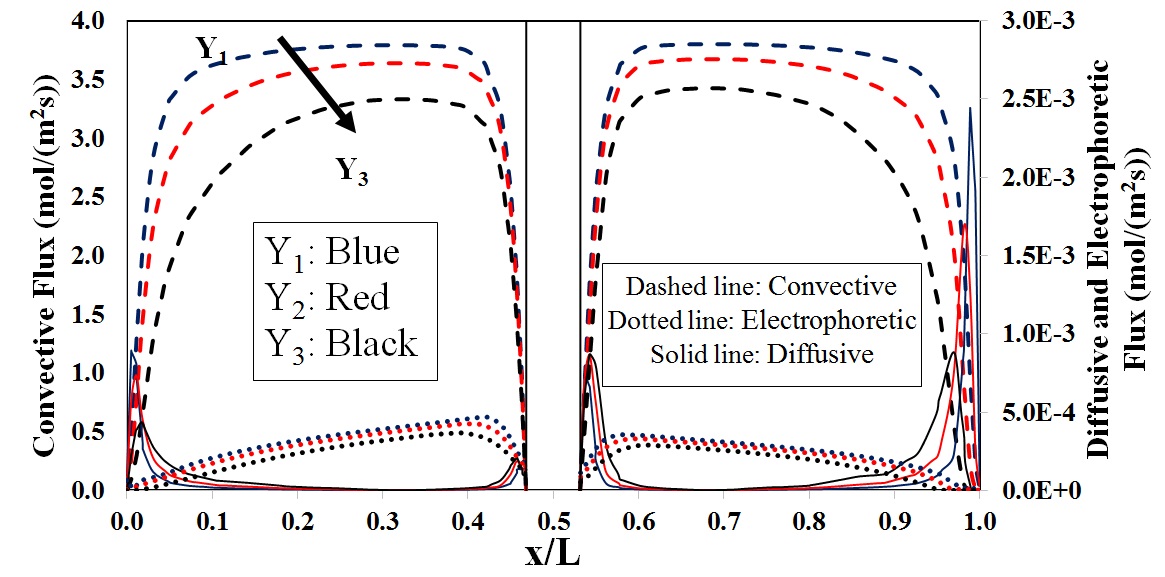
Diffusive, convective and electrophoretic flux prediciton for V(II)/V(V)
|
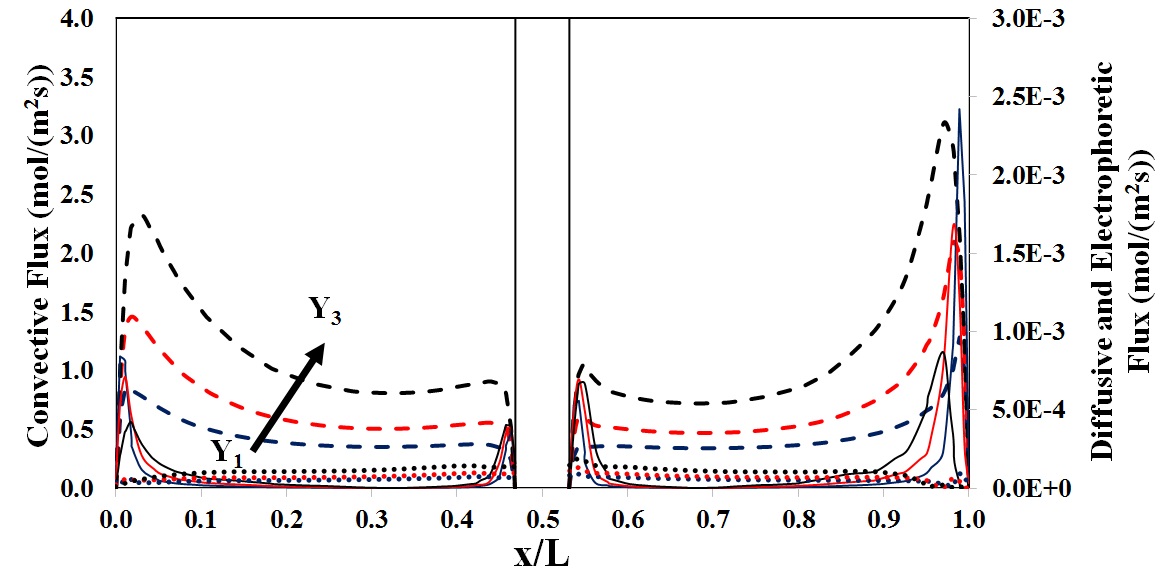
Diffusive, convective and electrophoretic flux prediciton for V(III)/V(IV) |
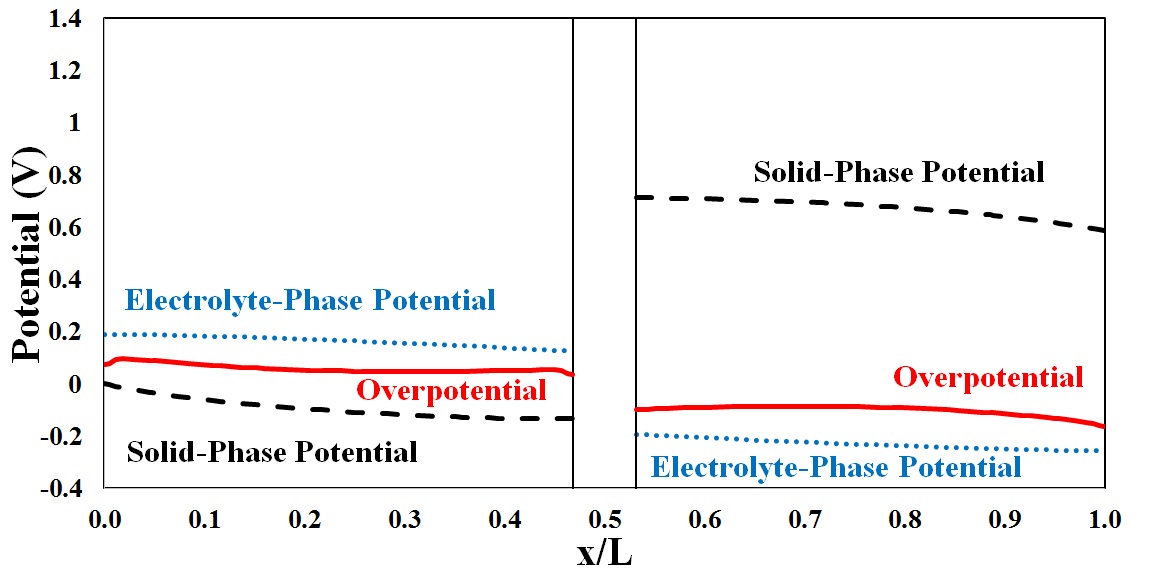
Potential distribution in the through-plane direction of
an operando VRFB |
e) Influence of Membrane Equivalent Weight and Reinforcement on Ionic Species Crossover in All-Vanadium Redox Flow Batteries
One of the major sources of lost capacity in all-vanadium redox flow batteries (VRFBs) is the undesired transport (usually called crossover) of water and vanadium ions through the ion-exchange membrane. In this work, an experimental assessment of the impact of ion-exchange membrane properties on vanadium ion crossover and capacity decay of VRFBs has been performed.
Two types of cationic membranes (non-reinforced and reinforced) with three equivalent weights of 800, 950 and 1100 g·mol−1 were investigated via a series of in situ performance and capacity decay tests along with ex situ vanadium crossover measurement (using UV/Vis spectroscopy) and membrane characterization.
|
Schematic of the experimental setup for measuring the crossover and capacity decay in VRFBs |
It is shown that for non-reinforced membranes, increasing the equivalent weight (EW) decreases the V(IV) permeability, but increases the area-specific resistance (ASR). This increase in ASR and decrease in V(IV) permeability was accompanied by increased through-plane membrane swelling. Comparing the non-reinforced with reinforced membranes, membrane reinforcement increases ASR, but V(IV) permeability decreases. It was also shown that there exists a monotonic correlation between the discharge capacity decay over long-term cycling and V(IV) permeability values. Thus, V(IV) permeability is considered a representative diagnostic for assessing the overall performance of a particular ion-exchange membrane with respect to capacity fade in a VRFB.
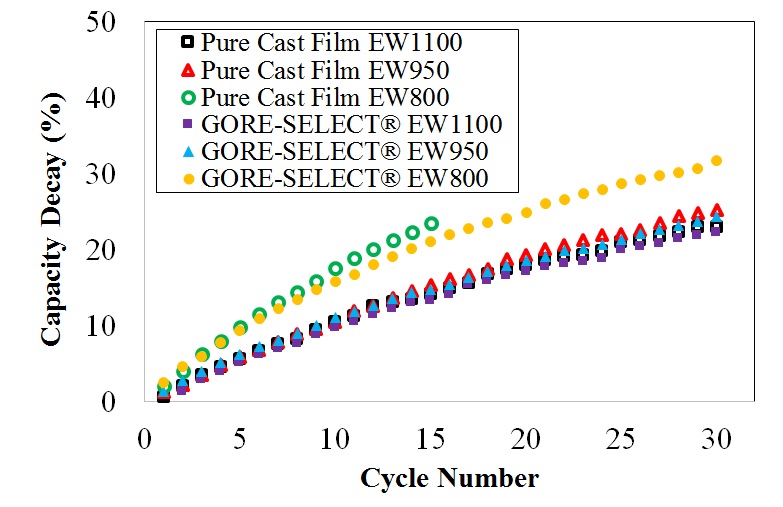
(a)
|
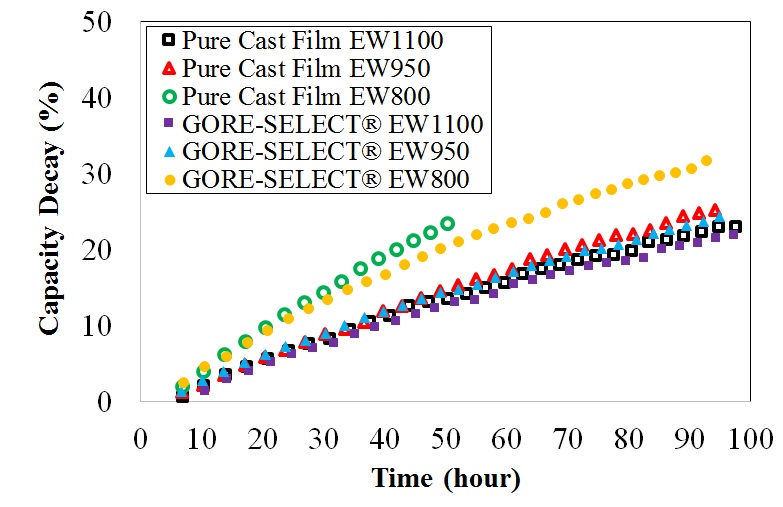
(b) |
Capacity decay for different ion-exchange membranes as a function of (a) cycle number and (b) time.
|
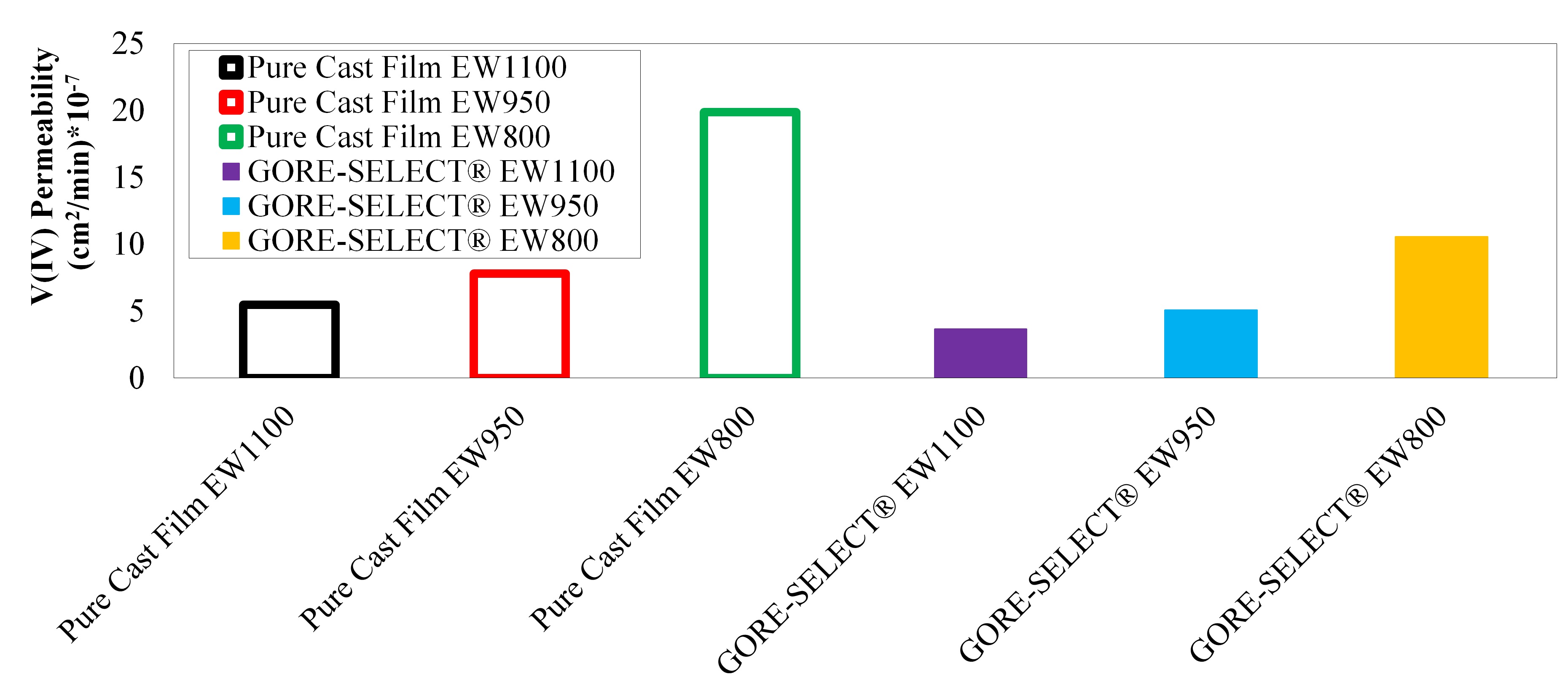
Measured permeability values for different membranes.
|
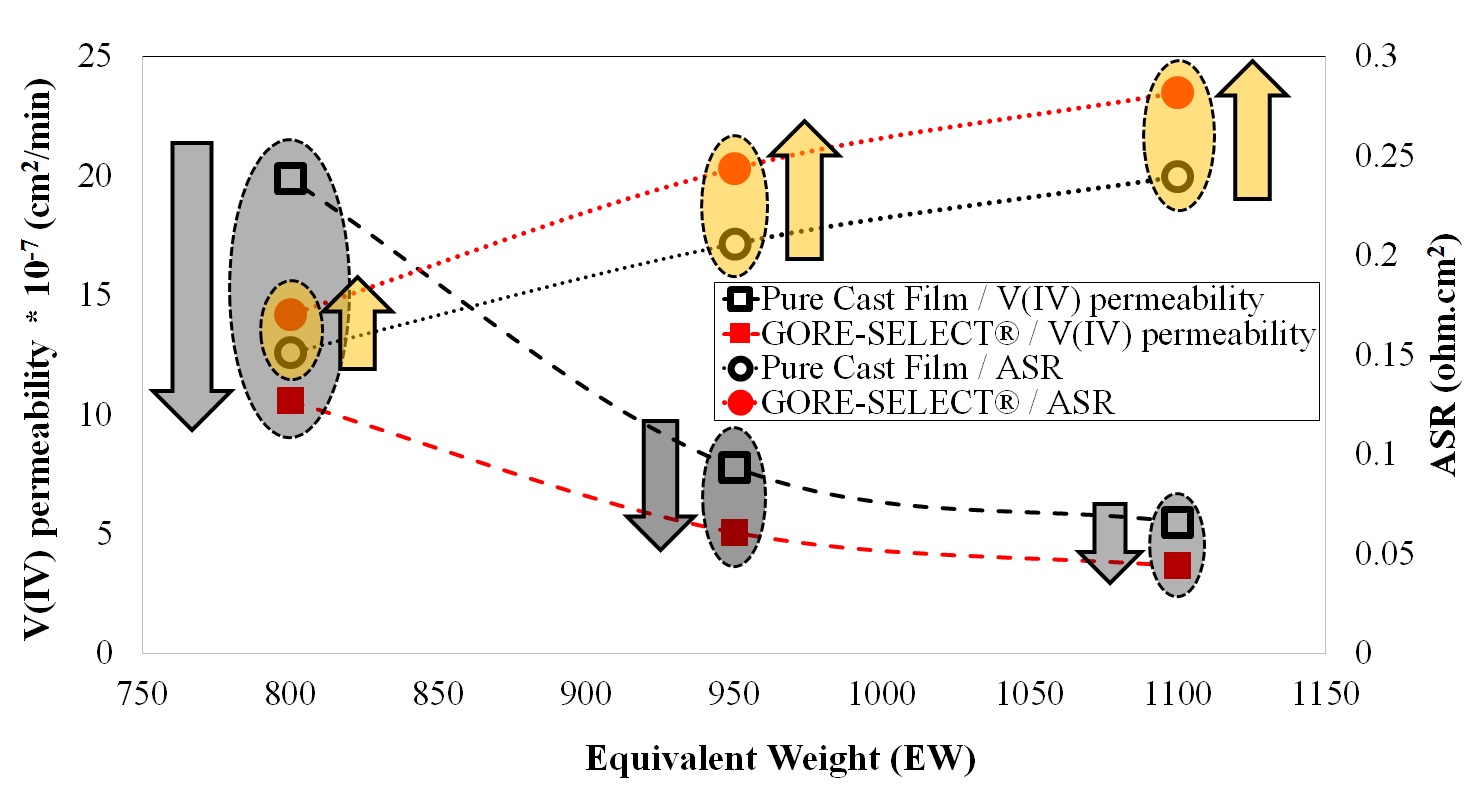
Correlation between equivalent weight with V(IV) permeability and ASR.
|
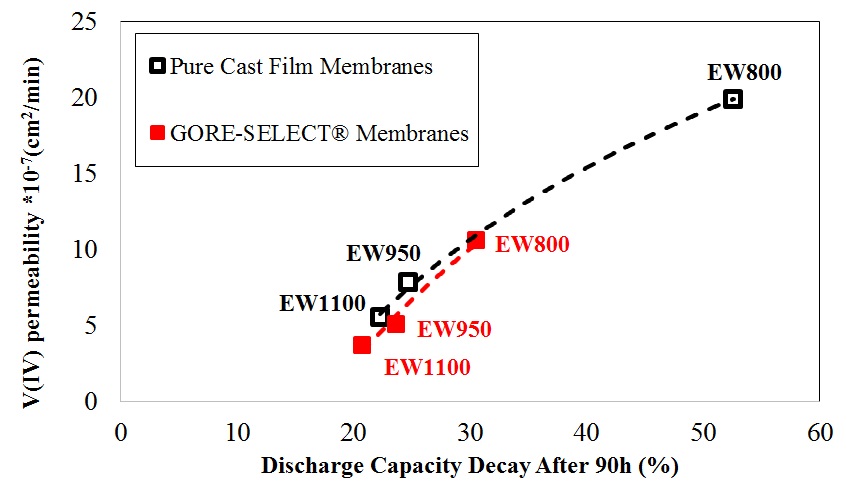
The discharge capacity decay at the end of cycling as function of vanadium V(IV) permeability. |
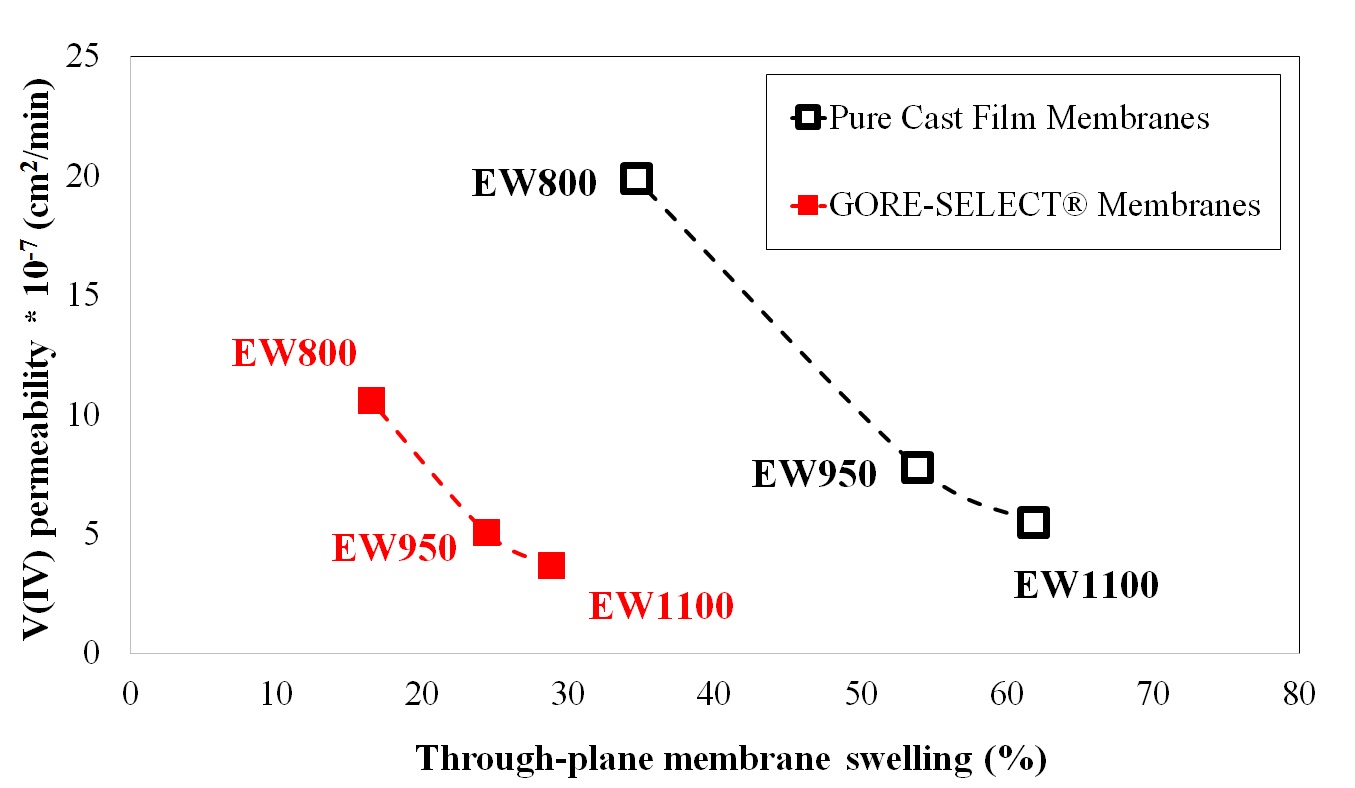
Correlation between V(IV) permeability and through-plane membrane swelling. |
Related patents:
- Yasser Ashraf Gandomi, M. M. Mench, “Novel Cell Designs for Passive Mitigation of Capacity Decay in Flow Batteries”, University of Tennessee Research Foundation’s Reference ID: 17156-03, Invention disclosure filed.
Related papers:
-
Yasser Ashraf Gandomi, D. S. Aaron, M. M. Mench, “Influence of Membrane Equivalent Weight and Reinforcement on Ionic Species Crossover in All-Vanadium Redox Flow Batteries”, Journal of Membranes 7 (2), 29 (2017).
- Yasser Ashraf Gandomi, D. S. Aaron, M. M. Mench, “Coupled Membrane Transport Parameters for Ionic Species in All-Vanadium Redox Flow Batteries”, Electrochimica Acta, 218, 174-190 (2016).
- Yasser Ashraf Gandomi, D. S. Aaron, T. A. Zawodzinski, M. M. Mench, “In-situ Potential Distribution Measurement and Validated Model for All-Vanadium Redox Flow Battery”, Journal of Electrochemical Society, Focus issue on Redox Flow Batteries - Reversible Fuel Cells, 163(1), A5188-A5201 (2016).
- Yasser Ashraf Gandomi, T. A. Zawodzinski, M. M. Mench, “Concentrated Solution Model of Transport in All Vanadium Redox Flow Battery Membrane Separator”, ECS Transactions, 61(13), 23-32 (2014).
back to top
3. Electrochemical Sensors (ES):
Developing highly sensitive portable sensors for early detection of the chemical warfare agents (CWAs) is of a great importance.
a) Toxic gas detection by laser direct written electrochemical sensors with a pulsed probing current
In this work, two configurations of carbon and copper-coated laser induced interdigitated microelectrode sensors were developed. Dimethyl methylphosphonate (DMMP) is used as the simulant representing Sarin gas morphology. The sensors were exposed to DMMP at ambient and an elevated temperature (60 C) and were subjected to a pulsed voltage excitation protocol.
|
Schematic of the fs laser direct writing of sensor on a PI sheet
|
Related papers:
- Yasser Ashraf Gandomi, S. Wang, V. G. Venkata, Y. Yu, S. Chakraborty, A. Hu, M. M. Mench, “Fast Detection of Toxic Gas by Laser Direct Written Electrochemical Sensors with a Pulsed Probing Current”, Submitted to Journal of Sensors and Actuators B (Chemical) (May 25, 2017).
Related patents:
- Yasser Ashraf Gandomi, S. Chakraborty, M. M. Mench, “Electrochemical Sensor for Simultaneous Detection of Multiple Contaminants”, University of Tennessee Research Foundation’s Reference ID: 16014-03, filed May 2015, Patent pending.
back to top
4. Multilevel Inverters (MIs)
back to top
|
|